2 Decomposers in the Tundra: Unsung Arctic Heroes
In the Arctic's harsh environment, the critical role of 2 decomposers in the tundra often goes unnoticed, yet these organisms are essential for nutrient cycling and ecosystem health. The fungi kingdom, a diverse group of eukaryotic organisms, represents one such decomposer, breaking down organic material and releasing vital nutrients back into the soil. Climate change, a global phenomenon, significantly impacts the activity of these decomposers, influencing the rate at which they process organic matter. The Arctic Council, an intergovernmental forum, acknowledges the importance of understanding these biological processes to assess the broader environmental changes occurring in this fragile biome. Specific bacterial species, often studied using microbial ecology techniques, constitute another crucial group of decomposers that thrive in the cold tundra soils.
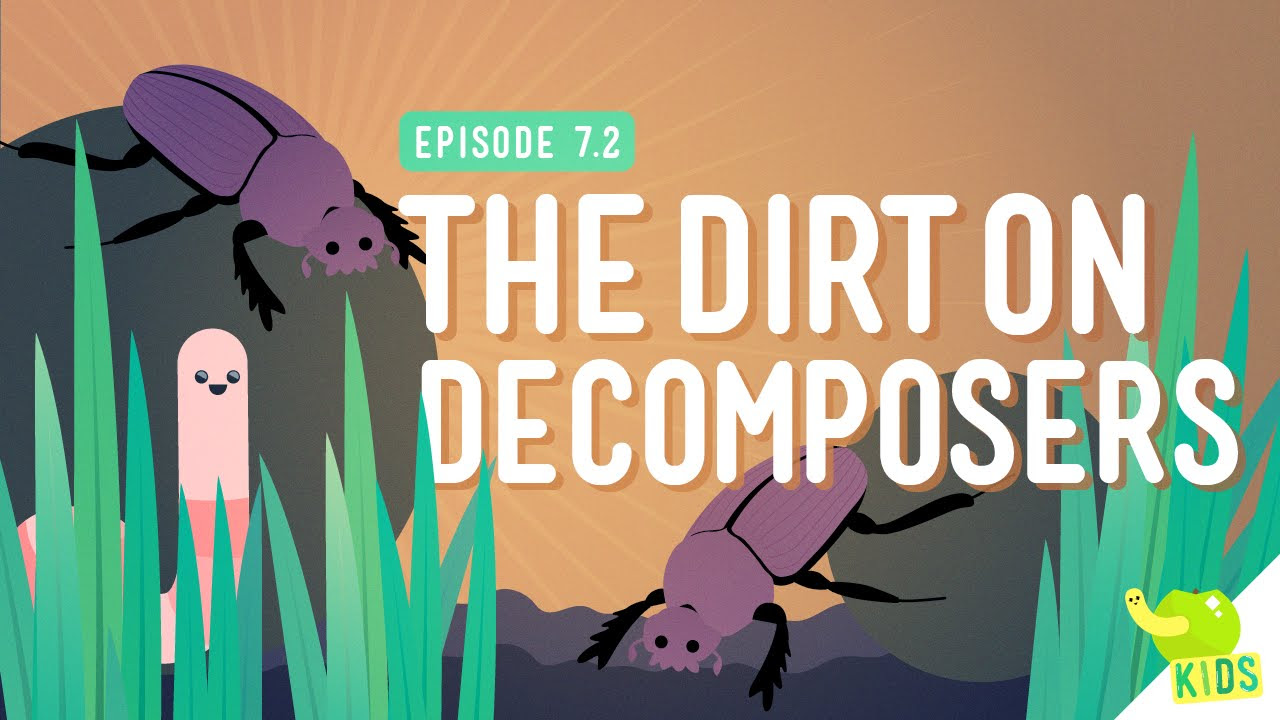
Image taken from the YouTube channel Crash Course Kids , from the video titled The Dirt on Decomposers: Crash Course Kids #7.2 .
Unveiling the Secrets of Tundra Decomposition
Decomposition, the breakdown of dead organic matter into simpler compounds, is a fundamental ecological process.
It's the engine that drives nutrient recycling and carbon cycling in nearly every ecosystem on Earth.
In the seemingly barren and harsh environment of the tundra, decomposition plays an outsized role, shaping the landscape and influencing global climate patterns.
This process, often overlooked, is critical for understanding the delicate balance of this fragile environment.
The Importance of Decomposition in the Tundra
Within the tundra ecosystem, decomposition underpins the entire food web.
It releases essential nutrients locked within dead plants and animals, making them available for uptake by the sparse vegetation.
Without decomposition, the limited nutrients in the tundra soil would quickly be exhausted, crippling plant growth and impacting the animals that depend on them.
Nutrient Cycling and the Carbon Cycle in Cold Environments
Decomposition is inextricably linked to both nutrient and carbon cycles.
The breakdown of organic matter releases vital elements like nitrogen, phosphorus, and potassium, which are essential for plant growth.
Simultaneously, decomposition is a key component of the carbon cycle.
It releases carbon dioxide (CO2) and, under certain conditions, methane (CH4), both potent greenhouse gases, into the atmosphere.
In cold environments like the tundra, these cycles are particularly sensitive to changes in temperature and moisture.
Even small shifts in these factors can drastically alter decomposition rates, impacting both nutrient availability and greenhouse gas emissions.
Unique Tundra Characteristics: Permafrost and the Active Layer
The tundra presents unique challenges and opportunities for decomposition due to its defining characteristics: permafrost and the active layer.
Permafrost, the permanently frozen ground, acts as a barrier, restricting decomposition by limiting microbial activity and nutrient availability.
The active layer, the surface layer that thaws seasonally, becomes the primary zone of decomposition.
Its thinness and short growing season constrain the overall rate of decay.
However, with climate change, permafrost thaw is accelerating, creating new areas for decomposition and potentially releasing vast stores of previously frozen carbon.
These unique features demand specific approaches to studying and understanding decomposition processes in the tundra.
The Unsung Heroes: Key Decomposers of the Tundra
Decomposition in the tundra is not a solo act; it's a complex performance orchestrated by a diverse cast of organisms, each playing a crucial role in breaking down organic matter and recycling nutrients.
While often unseen and uncelebrated, these decomposers are the unsung heroes of the Arctic, quietly driving the engine of this fragile ecosystem.
Fungi and bacteria form the core of this decomposition crew, but other organisms also contribute, adding layers of complexity to the process.
Fungi: The Filamentous Foragers
Fungi are arguably the most important decomposers in the tundra.
Their filamentous structure allows them to penetrate and colonize dead plant material, accessing nutrients that would otherwise be unavailable.
They produce a wide array of enzymes capable of breaking down complex polymers like cellulose and lignin, the tough components of plant cell walls.
This enzymatic action unlocks essential nutrients, making them accessible to other organisms and the surrounding environment.
Bacteria: The Microscopic Movers
Bacteria also play a vital role in tundra decomposition, often working in tandem with fungi.
They thrive in a wide range of conditions, including the cold, waterlogged environments common in the tundra.
Many bacteria specialize in breaking down specific types of organic matter, contributing to the overall efficiency of the decomposition process.
Some bacteria are even capable of breaking down more recalcitrant compounds that fungi struggle with.
The Supporting Cast: Springtails, Enchytraeids, and Nematodes
While fungi and bacteria handle the bulk of the decomposition work, other organisms also play significant roles.
Springtails (Collembola) are tiny arthropods that feed on fungi, bacteria, and decaying organic matter.
Their grazing activity helps to fragment organic material, increasing its surface area and making it more accessible to microbial decomposers.
Enchytraeids, small worms related to earthworms, also contribute to decomposition by feeding on decaying matter and mixing it with the soil.
Nematodes, microscopic roundworms, further contribute to the complexity of the soil food web, feeding on bacteria, fungi, and other nematodes.
This complex network of interactions enhances nutrient cycling and decomposition rates.
Coprinus psychromorbidus: A Cold-Adapted Specialist
Some decomposers have evolved specific adaptations to thrive in the harsh conditions of the tundra.
Coprinus psychromorbidus is a cold-adapted fungus that is particularly important in winter decomposition.
It can actively break down organic matter even under snow cover, when other decomposers are largely inactive.
This cold-tolerance allows C. psychromorbidus to contribute to year-round nutrient cycling.
Its importance highlights the specialization that enables decomposition to continue even in the face of extreme cold.
Environmental Orchestration: Factors Influencing Decomposition Rates
Decomposition rates in the tundra are not uniform; they are instead meticulously governed by a complex interplay of environmental factors.
These factors, ranging from the presence of permafrost to the dynamics of the active layer, dictate the pace at which organic matter is broken down and nutrients are recycled.
Understanding these controls is crucial for comprehending the tundra's role in global biogeochemical cycles, particularly in the context of ongoing climate change.
The Constraining Grip of Permafrost
Permafrost, the perennially frozen ground that underlies much of the tundra, exerts a profound influence on decomposition.
By maintaining extremely low temperatures, permafrost effectively inhibits the activity of decomposer organisms, such as fungi and bacteria.
This thermal constraint drastically slows down the breakdown of organic material.
In addition, permafrost acts as a physical barrier, preventing the downward migration of nutrients and restricting root growth.
This further limits the availability of essential elements for both decomposers and plants, thereby curtailing decomposition rates.
The Active Layer: A Stage for Decomposition
Above the permafrost lies the active layer, a zone of soil that thaws seasonally.
This layer serves as the primary site of decomposition in the tundra, where microbial activity is concentrated during the warmer months.
The thickness of the active layer, which varies depending on factors like snow cover, vegetation type, and soil characteristics, directly influences the amount of organic matter that can be processed annually.
Two key factors within the active layer—temperature and moisture—exert particularly strong control over decomposition rates.
Temperature's Pivotal Role
Temperature is a critical driver of microbial activity.
As temperatures rise within the active layer during the short Arctic summer, decomposers become more active.
This leads to an accelerated breakdown of organic matter.
However, the relatively short duration of warm temperatures limits the overall decomposition potential.
Even small increases in temperature due to climate change can have significant effects on decomposition rates.
Moisture's Complex Influence
Moisture availability plays a more nuanced role in regulating decomposition.
While adequate moisture is essential for microbial activity, excessive water can lead to waterlogged conditions and anaerobic decomposition.
Anaerobic decomposition, which occurs in the absence of oxygen, is a slower and less efficient process compared to aerobic decomposition.
It also leads to the production of methane (CH4), a potent greenhouse gas.
The balance between aerobic and anaerobic conditions, therefore, significantly influences the rate and products of decomposition.
Alaskan Tundra: A Case Study
The Alaskan tundra provides a compelling example of how these environmental factors interact to influence decomposition.
Across different regions of Alaska, variations in permafrost depth, active layer thickness, temperature regimes, and moisture levels result in a mosaic of decomposition rates.
For example, areas with deeper active layers and warmer summers exhibit faster decomposition rates compared to those with shallow active layers and colder conditions.
Furthermore, differences in vegetation type and soil properties contribute to spatial variability in decomposition across the Alaskan tundra.
These variations underscore the importance of considering the complex interplay of environmental factors when assessing the role of decomposition in the Arctic carbon cycle.
Nutrient and Carbon Crossroads: The Role of Decomposition in Ecosystem Cycles
The intricate dance between decomposition and biogeochemical cycles forms the very backbone of the tundra ecosystem.
It is a system where the breakdown of organic matter fuels plant life and, simultaneously, regulates the flow of carbon—a critical element in a world grappling with climate change.
Understanding this interplay is paramount to comprehending the tundra's sensitivity to environmental shifts and its potential impact on global climate dynamics.
Decomposition and the Nutrient Cycle: A Symbiotic Relationship
Decomposition is not merely a process of decay; it is a vital link in the tundra's nutrient cycle.
As decomposers work their magic on dead plant material, animal remains, and other organic detritus, they liberate essential nutrients that would otherwise remain locked away.
This released bounty—nitrogen, phosphorus, potassium, and a suite of other minerals—becomes available for uptake by plants, fueling their growth and sustaining the entire food web.
Without decomposition, the tundra would be a barren landscape, unable to support the life it currently harbors.
Nutrient Liberation: Fueling Tundra Plant Life
The process of decomposition is essentially a nutrient liberation strategy.
Plants need these elements to synthesize proteins, enzymes, and other vital biomolecules.
Through the action of decomposers, nutrients are converted from complex organic forms into simpler, inorganic forms that plants can readily absorb through their roots.
This nutrient recycling is particularly important in the tundra, where nutrient availability is inherently limited by low temperatures and slow decomposition rates.
Decomposition and the Carbon Cycle: A Delicate Balance
Decomposition also plays a pivotal role in the carbon cycle, influencing the balance of carbon storage and release in the tundra.
As organic matter is broken down, carbon is released into the atmosphere in the form of carbon dioxide (CO2) and methane (CH4).
The proportion of each gas released depends on whether the decomposition process occurs in the presence of oxygen (aerobic) or in its absence (anaerobic).
Aerobic decomposition primarily yields CO2, while anaerobic decomposition, common in waterlogged tundra soils, produces significant amounts of CH4, a far more potent greenhouse gas than CO2.
Carbon Release: A Double-Edged Sword
The release of CO2 and CH4 from decomposition has both ecological and climatic implications.
On one hand, the release of CO2 can stimulate plant growth, as CO2 is a key ingredient in photosynthesis.
However, the increased greenhouse gas concentration in the atmosphere contributes to global warming, which, in turn, further accelerates decomposition and creates a positive feedback loop.
The potential for large-scale carbon release from thawing permafrost, driven by accelerated decomposition, represents a significant concern for climate scientists.
Climate Change: A Tundra Transformation
The Arctic tundra, a landscape long characterized by its frozen grip, now stands at a critical juncture. The accelerating pace of climate change is not merely altering temperatures and precipitation patterns; it is fundamentally reshaping the very processes that govern this fragile ecosystem. Decomposition, once a slow and deliberate affair dictated by the constraints of a frozen world, is now undergoing a dramatic transformation, with far-reaching consequences for the tundra's carbon balance and the global climate system.
The Warming Arctic: A Catalyst for Change
Rising temperatures, perhaps the most visible manifestation of climate change in the Arctic, are having a profound impact on tundra decomposition. The increase in average annual temperatures extends the growing season, providing a longer window of opportunity for microbial activity and the breakdown of organic matter.
Warmer temperatures directly stimulate the metabolic rates of decomposers, accelerating the breakdown of plant litter, animal remains, and other organic detritus. This, in turn, releases nutrients and carbon into the ecosystem, setting off a cascade of ecological and biogeochemical changes.
Altered precipitation patterns, including shifts in the timing and intensity of rainfall and snowfall, also play a critical role. Changes in moisture levels influence soil moisture content, which directly impacts the activity of decomposers and the rate of decomposition. Furthermore, increased snow cover can insulate soils during winter, leading to warmer soil temperatures and sustained decomposition even during the colder months.
Permafrost Thaw: Unearthing the Arctic's Carbon Legacy
Perhaps the most significant consequence of climate change for tundra decomposition is the widespread thawing of permafrost. Permafrost, the permanently frozen ground that underlies much of the Arctic, contains vast stores of organic carbon accumulated over millennia.
As temperatures rise, this permafrost begins to thaw, exposing previously frozen organic matter to decomposition. This thawed organic matter, often rich in easily degradable compounds, becomes readily available to decomposers, leading to a dramatic increase in decomposition rates.
The accelerated decomposition of permafrost carbon is a major concern because it releases large quantities of carbon dioxide (CO2) and methane (CH4) into the atmosphere. These greenhouse gases contribute to global warming, creating a positive feedback loop that further accelerates permafrost thaw and decomposition.
Implications for the Carbon Balance and Global Climate
The altered decomposition rates driven by climate change have profound implications for the carbon balance of the tundra. The tundra, which has historically acted as a carbon sink, sequestering more carbon than it releases, is increasingly becoming a net source of carbon to the atmosphere.
The release of CO2 and CH4 from thawing permafrost and accelerated decomposition is contributing to the overall increase in greenhouse gas concentrations in the atmosphere, exacerbating global warming and climate change.
The magnitude of this carbon release is uncertain and depends on a variety of factors, including the rate of permafrost thaw, the composition of the organic matter, and the environmental conditions that influence decomposition. However, the potential for large-scale carbon release from the tundra represents a significant threat to global climate stability.
Furthermore, the altered decomposition rates can affect the availability of nutrients in the tundra ecosystem, potentially impacting plant growth and the composition of plant communities. Changes in plant communities can, in turn, influence decomposition rates, creating complex interactions that are difficult to predict.
Understanding the impact of climate change on tundra decomposition is essential for predicting future climate change scenarios and developing strategies to mitigate its effects. Further research and monitoring efforts are needed to better understand the complex interactions between climate change, permafrost thaw, decomposition, and the carbon cycle in the Arctic tundra.
Pathways of Decay: Aerobic vs. Anaerobic Decomposition
The decomposition of organic matter in the tundra is not a monolithic process. Rather, it unfolds along distinct pathways, primarily dictated by the presence or absence of oxygen. These pathways—aerobic and anaerobic decomposition—exhibit fundamental differences in their mechanisms, rates, and end-products, ultimately shaping the tundra's carbon dynamics and its contribution to greenhouse gas emissions.
Aerobic Decomposition: The Breath of Life
Aerobic decomposition, as the name suggests, occurs in the presence of oxygen. It's the dominant mode of decay in well-drained tundra soils where oxygen readily penetrates.
Under these conditions, decomposers, primarily bacteria and fungi, utilize oxygen to break down complex organic molecules into simpler compounds, releasing energy in the process. This process yields carbon dioxide (CO2) as a primary byproduct, along with water and mineral nutrients.
Aerobic decomposition is generally a more efficient process than its anaerobic counterpart, leading to a relatively faster breakdown of organic matter. This rapid turnover of nutrients can be crucial for plant growth during the short Arctic growing season.
Anaerobic Decomposition: When Oxygen is Scarce
In contrast, anaerobic decomposition occurs in the absence of oxygen. This is typical in waterlogged areas, such as bogs and wetlands, where the diffusion of oxygen into the soil is severely limited.
Under these anaerobic conditions, a different suite of microorganisms, including anaerobic bacteria and archaea, takes center stage. These organisms employ alternative electron acceptors, such as sulfate or carbon dioxide, to break down organic matter.
This process is significantly slower and less efficient compared to aerobic decomposition, resulting in the accumulation of partially decomposed organic matter.
A critical difference lies in the production of methane (CH4), a potent greenhouse gas, as a major byproduct of anaerobic decomposition.
Contrasting Environments: Waterlogged vs. Well-Drained
The contrasting conditions of waterlogged versus well-drained soils profoundly influence the balance between aerobic and anaerobic decomposition. Well-drained soils, typically found on higher ground or slopes, facilitate oxygen diffusion, promoting aerobic decomposition and CO2 release.
These areas are often characterized by relatively faster nutrient cycling and plant growth.
Conversely, waterlogged soils, prevalent in low-lying areas and permafrost-affected regions, restrict oxygen availability, favoring anaerobic decomposition and methane production.
The rise in water table levels due to increased precipitation and permafrost thaw is expanding the spatial extent of anaerobic environments in the tundra, with potentially significant implications for greenhouse gas emissions.
Greenhouse Gas Implications: Methane's Role
The varying impacts of aerobic and anaerobic decomposition on greenhouse gas emissions are a central concern in the context of climate change. While aerobic decomposition primarily releases CO2, a well-known greenhouse gas, anaerobic decomposition produces both CO2 and methane (CH4).
Methane is a significantly more potent greenhouse gas than CO2 over shorter timescales, with a global warming potential many times higher. The production of methane under anaerobic conditions represents a significant positive feedback loop, accelerating climate change.
As the tundra warms and permafrost thaws, the expansion of waterlogged areas promotes anaerobic decomposition and, consequently, increased methane emissions.
The complex interplay between aerobic and anaerobic processes in the tundra underscores the need for a nuanced understanding of decomposition pathways. This understanding is vital for predicting the future carbon balance of the Arctic and mitigating the effects of climate change.
Detritus Demystified: Fueling the Tundra Decomposers
The decomposition process, so central to the tundra ecosystem, hinges on a fundamental resource: detritus. Understanding detritus – its nature, composition, and influence – is crucial for deciphering the dynamics of decomposition in this fragile environment.
Defining Detritus: The Foundation of Decomposition
Detritus, in its broadest sense, encompasses non-living organic matter. This includes everything from dead plant material and animal remains to fecal matter and other organic debris.
In the tundra, detritus serves as the primary source of energy and nutrients for decomposers. These decomposers, in turn, drive nutrient cycling and carbon flow within the ecosystem.
Without a consistent supply of detritus, the entire decomposition process would grind to a halt, disrupting the delicate balance of the tundra.
The Composition of Tundra Detritus: A Varied Feast
The specific composition of detritus varies depending on the location, season, and dominant plant and animal species.
Plant litter, consisting of dead leaves, stems, and roots, forms a significant portion of tundra detritus.
Animal remains, though often less abundant, also contribute essential nutrients.
Additionally, the presence of organic matter from other sources, such as microbial biomass and dissolved organic carbon, further diversifies the detrital pool.
Plant Litter: A Dominant Component
The slow growth rates and recalcitrant nature of many tundra plants contribute to the accumulation of substantial amounts of plant litter.
Species like dwarf shrubs, sedges, and mosses create a dense layer of dead organic material that slowly decomposes over time.
The chemical composition of this plant litter, particularly its lignin and cellulose content, plays a vital role in determining its decomposition rate.
Animal Remains: A Nutrient-Rich Source
While animal remains may constitute a smaller fraction of the total detritus, they can be a critical source of readily available nutrients, such as nitrogen and phosphorus.
Decomposition of animal carcasses, fecal matter, and shed exoskeletons provides a pulse of nutrients that can stimulate microbial activity and plant growth.
Detritus Availability and Quality: Controlling the Pace of Decay
The availability and quality of detritus are major determinants of decomposition rates in the tundra. These factors dictate the rate at which decomposers can access and process organic matter, impacting nutrient release and carbon cycling.
The Influence of Availability
A consistent supply of detritus is essential for sustaining a healthy population of decomposers.
Factors such as plant productivity, herbivore activity, and disturbance events can influence the amount of detritus entering the decomposition cycle.
In areas with limited detrital input, decomposition rates may be significantly slower, leading to nutrient limitations and reduced plant growth.
The Significance of Quality
The chemical composition of detritus profoundly affects its decomposability. Substrates with high carbon-to-nitrogen ratios, for example, decompose more slowly than those with lower ratios.
The presence of recalcitrant compounds, such as lignin and tannins, can further inhibit decomposition by making organic matter less accessible to microbial enzymes.
Conversely, detritus rich in labile compounds, such as sugars and proteins, is readily decomposed, fueling rapid nutrient release and microbial growth.
Guardians of Knowledge: Research and Monitoring Initiatives
The study of tundra decomposition, and its intricate link to global climate patterns, relies heavily on the dedicated efforts of various research groups and monitoring initiatives. These guardians of knowledge tirelessly work to unravel the complexities of this fragile ecosystem.
Their investigations provide critical insights into the current state of the tundra and project potential future changes.
Leading the Charge: Key Research Organizations
Several prominent organizations stand at the forefront of tundra research. They contribute significantly to our understanding of decomposition processes.
University research groups, often operating with substantial grant funding and specialized equipment, form the backbone of scientific inquiry. These groups conduct field experiments, laboratory analyses, and modeling studies. They address fundamental questions about tundra ecology.
The Arctic Research Consortium of the United States (ARCUS) plays a crucial role in coordinating and supporting arctic research efforts. ARCUS facilitates collaboration among researchers, promotes data sharing, and disseminates findings to a wider audience.
The International Tundra Experiment (ITEX) is a global network of research sites. It focuses on monitoring the impacts of climate change on tundra ecosystems.
ITEX employs standardized methodologies to collect data on plant growth, decomposition rates, and other key ecological variables. This allows for comparative analyses across different tundra regions.
University Research Groups: Deep Dives into Decomposition
University-based research teams bring specialized expertise to the study of tundra decomposition. They conduct in-depth investigations of specific aspects of the process.
For instance, some groups focus on the microbial communities responsible for breaking down organic matter. They analyze the diversity and function of bacteria and fungi in tundra soils.
Other teams investigate the impact of permafrost thaw on decomposition rates. They measure the release of carbon dioxide and methane from thawing soils.
Still others examine the role of different plant species in influencing decomposition rates. They study the chemical composition of plant litter and its effect on microbial activity.
ARCUS: Facilitating Collaboration and Knowledge Sharing
ARCUS serves as a vital hub for arctic research. It brings together researchers from diverse disciplines and institutions.
ARCUS fosters collaboration by organizing workshops, conferences, and other networking events. It also supports the development of research infrastructure and data management systems.
By promoting data sharing and open access, ARCUS ensures that research findings are readily available to the scientific community. This accelerates the pace of discovery and facilitates more informed decision-making.
ITEX: A Global Perspective on Tundra Change
ITEX provides a valuable global perspective on the impacts of climate change on tundra ecosystems. It employs standardized monitoring protocols across a network of research sites.
This allows for direct comparisons of decomposition rates and other ecological variables across different tundra regions. ITEX provides insights into the extent to which tundra ecosystems are responding to climate change.
The long-term monitoring data collected by ITEX is invaluable for tracking trends over time. It also helps to distinguish between natural variability and climate-driven changes.
By combining local expertise with a global framework, ITEX contributes to a more comprehensive understanding of the challenges facing tundra ecosystems.
Contributions to Understanding Tundra Decomposition
These research groups and monitoring initiatives have made significant contributions to our understanding of tundra decomposition. They have shed light on the factors controlling decomposition rates, the role of decomposers in nutrient cycling, and the impacts of climate change on these processes.
Their findings have helped to refine models of the tundra carbon cycle. They improve our ability to predict the future trajectory of this critical ecosystem.
The insights gained from these studies are essential for informing policy decisions. They help to mitigate the impacts of climate change on the tundra. Continued support for these research efforts is crucial for ensuring the long-term health and stability of this important ecosystem.
Tools of the Trade: Unraveling Decomposition Through Science
Understanding the intricate process of tundra decomposition requires a multifaceted approach, leveraging a diverse array of scientific methodologies. These tools of the trade allow researchers to delve into the complex interactions between soil, microbes, climate, and vegetation that govern the fate of organic matter in this fragile ecosystem.
From meticulous soil analysis to cutting-edge molecular techniques, these methods provide critical insights into the mechanisms driving decomposition and its response to environmental change.
Soil Analysis: A Window into Tundra Chemistry
Soil analysis forms the cornerstone of many tundra decomposition studies. By carefully examining soil samples, scientists can gain valuable information about nutrient availability, microbial activity, and the overall chemical environment that shapes decomposition rates.
These analyses typically involve measuring key parameters such as pH, organic matter content, nitrogen and phosphorus levels, and the abundance of various enzymes involved in decomposition.
Variations in these parameters can provide clues about the factors limiting decomposition in different tundra environments.
Probing Nutrient Availability
The availability of essential nutrients, such as nitrogen and phosphorus, plays a crucial role in regulating microbial growth and decomposition rates. Soil analysis techniques can quantify the concentrations of these nutrients in different forms, providing insights into their accessibility to decomposers.
For example, measurements of ammonium and nitrate levels reveal the amount of readily available nitrogen, while assessments of organic phosphorus content indicate the potential for future nutrient release through microbial activity.
Assessing Microbial Activity
Soil analysis can also provide indirect measures of microbial activity. Enzyme assays, for instance, quantify the activity of specific enzymes involved in the breakdown of organic matter, such as cellulases and chitinases.
Higher enzyme activities generally indicate greater microbial decomposition potential.
The presence and abundance of certain biomarkers, such as phospholipid fatty acids (PLFAs), can also provide information about the composition and activity of the microbial community.
Isotope Tracing: Following the Carbon and Nutrient Trail
Isotope tracing is a powerful technique used to track the movement of carbon and nutrients through the tundra ecosystem. By introducing stable or radioactive isotopes into the soil or plant litter, researchers can follow their incorporation into microbial biomass, their release as decomposition products, and their uptake by plants.
This approach provides valuable information about the pathways and rates of nutrient cycling and carbon flow.
It illuminates the fate of organic matter as it decomposes.
Unveiling Carbon Dynamics
Stable isotopes of carbon, such as 13C, are commonly used to trace the flow of carbon through the tundra ecosystem. By labeling plant litter with 13C, researchers can track its decomposition over time and quantify the amount of carbon released as CO2 or incorporated into microbial biomass.
This approach helps to determine the relative importance of different decomposition pathways and the overall efficiency of carbon cycling.
Tracing Nutrient Pathways
Isotopes of nitrogen and phosphorus can be used to trace the movement of these essential nutrients through the tundra ecosystem. By labeling plant litter or soil microbes with 15N or 33P, researchers can track their uptake by plants and their release as decomposition products.
This approach provides insights into the mechanisms of nutrient cycling and the factors regulating nutrient availability.
Microbial Community Analysis and DNA Sequencing: Decoding the Decomposers
Microbial community analysis and DNA sequencing have revolutionized our understanding of the diversity and function of tundra decomposers. These techniques allow researchers to identify the different types of bacteria, fungi, and other microorganisms present in tundra soils and to assess their roles in decomposition.
DNA sequencing provides a comprehensive inventory of the microbial community. It identifies the genes encoding key enzymes involved in decomposition.
Unraveling Microbial Diversity
Techniques such as 16S rRNA gene sequencing for bacteria and archaea, and ITS sequencing for fungi, allow researchers to identify the different types of microorganisms present in tundra soils.
These analyses have revealed a surprisingly diverse microbial community, with hundreds or even thousands of different species coexisting in a single soil sample.
Assessing Microbial Function
Metagenomics, the study of the collective genomes of microorganisms in a sample, can provide insights into the functional potential of the microbial community.
By analyzing the genes present in the metagenome, researchers can identify the enzymes and metabolic pathways involved in decomposition. This helps to understand how microbial communities respond to environmental changes.
Respiration Measurements: Quantifying Decomposition Activity
Respiration measurements provide a direct assessment of decomposition activity by quantifying the amount of carbon dioxide (CO2) released from soil. CO2 is a primary byproduct of microbial respiration. Its measurement offers insights into the overall rate of organic matter breakdown.
These measurements can be conducted in the laboratory using soil incubations or in the field using automated chambers.
Laboratory Incubations
Laboratory incubations involve placing soil samples in sealed containers and measuring the accumulation of CO2 over time. This approach allows for precise control of environmental conditions, such as temperature and moisture.
It helps to isolate the effects of individual factors on decomposition rates.
Field Chamber Measurements
Field chamber measurements involve placing chambers over the soil surface and measuring the concentration of CO2 inside the chamber over time. This approach provides a more realistic assessment of decomposition rates under natural conditions.
Automated chambers can continuously monitor CO2 fluxes. They provide valuable data on the temporal dynamics of decomposition.
Video: 2 Decomposers in the Tundra: Unsung Arctic Heroes
FAQs: 2 Decomposers in the Tundra
What are the two main decomposers discussed in the context of the Arctic tundra?
The article highlights fungi and bacteria as significant decomposers in the tundra environment. These 2 decomposers in the tundra play a critical role in breaking down organic matter.
Why are decomposers so important in the tundra ecosystem?
Decomposers are essential because they recycle nutrients locked within dead plants and animals. Without these 2 decomposers in the tundra breaking down organic material, nutrients would remain unavailable, hindering new plant growth.
What challenges do decomposers face in the tundra?
The cold temperatures and short growing seasons in the tundra slow down the decomposition process. This makes the work of these 2 decomposers in the tundra more difficult and protracted.
How does decomposition in the tundra differ from decomposition in warmer climates?
Decomposition in warmer climates is generally faster due to optimal temperatures and moisture. The slow pace of decomposition by 2 decomposers in the tundra leads to a build-up of organic matter, contributing to the formation of peat and permafrost.
So, next time you think of the Arctic, remember it's not just polar bears and penguins (well, not penguins in the Arctic!). It's also got a thriving, albeit hidden, community working tirelessly to keep everything in balance. Give a little shout-out to the real MVPs – the 2 decomposers in the tundra, like fungi and bacteria, that are essential for life as we know it, even in the coldest corners of the world.