Crest of a Wave: Ocean Dynamics & Coastal Safety
The National Oceanic and Atmospheric Administration (NOAA) monitors ocean conditions, and their data indicates that the crest of a wave often marks the most dangerous point for coastal areas. Understanding the physics of wave formation, a key area of study in ocean dynamics, is crucial for predicting when these crests will reach hazardous heights. Rip currents, intensified by wave action, frequently form near the shore, posing a significant threat to swimmers caught near the crest of a wave. Advanced wave forecasting models are employed to predict these conditions, aiding in the implementation of coastal safety measures and timely warnings to the public.
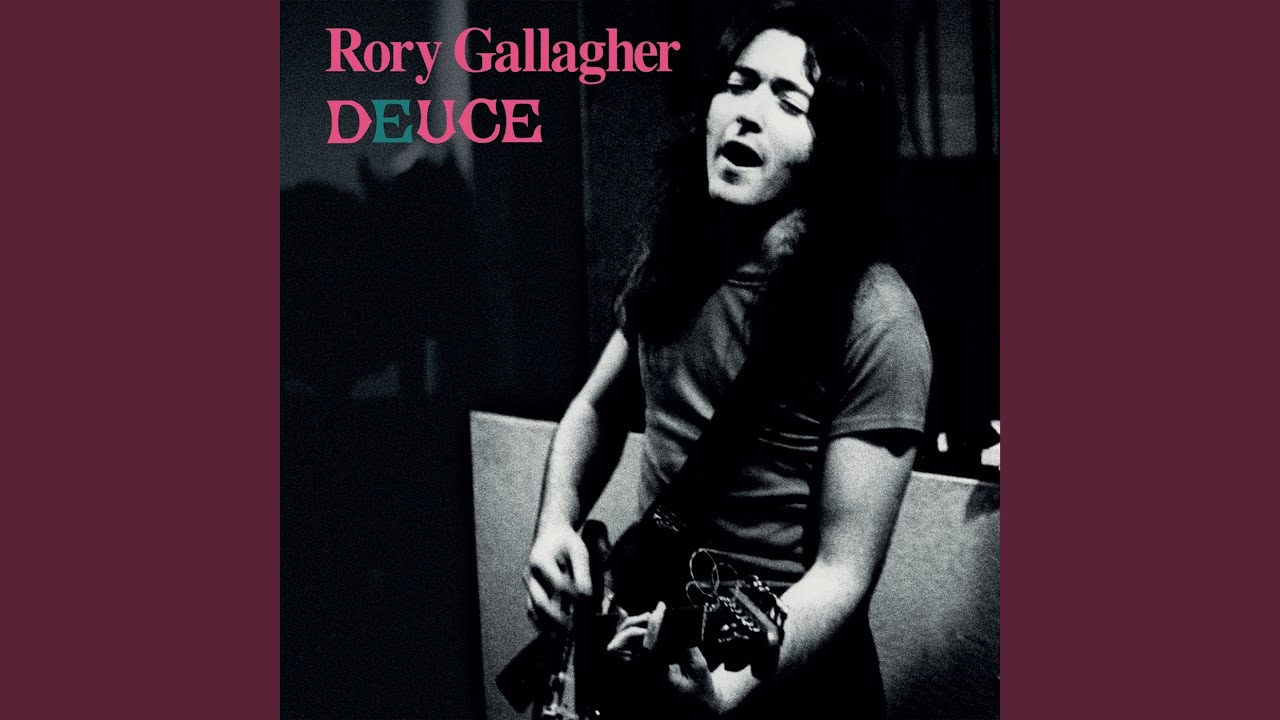
Image taken from the YouTube channel Rory Gallagher , from the video titled Crest Of A Wave .
Ocean waves are the sculptors of our coastlines, the tireless energy that ceaselessly reshapes the interface between land and sea. They are far more than just a picturesque backdrop; they are a primary force responsible for the dynamic environments we call coastlines.
From the gentle lapping of waves on a sandy beach to the destructive power of storm surges, understanding these complex phenomena is crucial for effective coastal management. We must grasp the intricacies of wave behavior.
The Unrelenting Power of Ocean Waves
Ocean waves are a manifestation of energy transfer, primarily from wind to the water's surface. This energy, once imparted, travels vast distances across the open ocean before finally expending itself upon reaching the shore.
Their impact is multifaceted. Waves erode cliffs, deposit sediment, create beaches, and shape the very contours of coastal landscapes.
This constant interplay between wave energy and coastal geology drives the evolution of coastlines over time. Ignoring this force is akin to ignoring the heartbeat of our planet's coastal zones.
Coastal Planning and Risk Mitigation: Why Understanding Waves Matters
Comprehending wave behavior is not merely an academic exercise; it is a fundamental necessity for responsible coastal planning and effective risk mitigation.
We need to understand how waves interact with coastal structures, how they contribute to erosion, and how they can lead to devastating floods.
By understanding wave dynamics, we can design coastal defenses that work with nature, rather than against it. This can lead to more sustainable and resilient coastal communities. Informed coastal management saves lives and protects valuable resources.
Predicting Coastal Erosion and Flooding
One of the most critical applications of wave dynamics lies in the prediction of coastal erosion and flooding.
By combining our knowledge of wave characteristics, coastal topography, and climate patterns, scientists can develop sophisticated models that forecast the impact of waves on coastal areas.
These models can help identify vulnerable regions, assess the risk of erosion and flooding, and inform the development of mitigation strategies.
For example, wave models can be used to predict the extent of coastal inundation during a storm surge, allowing authorities to issue timely warnings and evacuate residents.
This predictive capability is essential for protecting lives, property, and the environment in the face of increasingly frequent and intense coastal hazards.
Ocean waves are the sculptors of our coastlines, the tireless energy that ceaselessly reshapes the interface between land and sea. They are far more than just a picturesque backdrop; they are a primary force responsible for the dynamic environments we call coastlines.
From the gentle lapping of waves on a sandy beach to the destructive power of storm surges, understanding these complex phenomena is crucial for effective coastal management. We must grasp the intricacies of wave behavior.
The Unrelenting Power of Ocean Waves
Ocean waves are a manifestation of energy transfer, primarily from wind to the water's surface. This energy, once imparted, travels vast distances across the open ocean before finally expending itself upon reaching the shore.
Their impact is multifaceted. Waves erode cliffs, deposit sediment, create beaches, and shape the very contours of coastal landscapes.
This constant interplay between wave energy and coastal geology drives the evolution of coastlines over time. Ignoring this force is akin to ignoring the heartbeat of our planet's coastal zones.
Coastal Planning and Risk Mitigation: Why Understanding Waves Matters
Comprehending wave behavior is not merely an academic exercise; it is a fundamental necessity for responsible coastal planning and effective risk mitigation.
We need to understand how waves interact with coastal structures, how they contribute to erosion, and how they can lead to devastating floods.
By understanding wave dynamics, we can design coastal defenses that work with nature, rather than against it. This can lead to more sustainable and resilient coastal communities. Informed coastal management saves lives and protects valuable resources.
Predicting Coastal Erosion and Flooding
One of the most critical applications of wave dynamics lies in the prediction of coastal erosion and flooding.
By combining our knowledge of wave characteristics, coastal topography, and climate patterns, scientists can develop sophisticated models that forecast the impact of waves on coastal areas.
These models can help identify vulnerable regions, assess the risk of erosion and flooding, and inform the development of mitigation strategies.
For example, wave models can be used to predict the extent of coastal inundation during a storm surge, allowing authorities to issue timely warnings and evacuate residents.
This predictive capability is essential for protecting lives, property, and the environment in the face of increasingly frequent and intense coastal hazards.
Unveiling Wave Characteristics: Height, Wavelength, and Period
To truly understand the impact of ocean waves, we must first dissect their fundamental characteristics. These parameters – wave height, wavelength, and wave period – are the building blocks of wave dynamics.
They dictate how waves behave, how they interact with coastal environments, and the amount of energy they carry. Understanding these elements is critical for predicting and mitigating coastal hazards.
Wave Height: A Measure of Energy
Wave height is perhaps the most intuitive wave characteristic. It is defined as the vertical distance between the crest (the highest point) and the trough (the lowest point) of a wave.
Wave height is typically measured in meters or feet and is a direct indicator of the wave's energy content.
Wave height can be measured using a variety of methods. Traditionally, visual observation from ships or coastal stations was common. However, modern techniques are more precise.
Wave buoys, equipped with sensors that record the rise and fall of the water surface, are now widely used. Satellite altimeters also provide valuable data on wave height over vast ocean areas.
The relationship between wave height and wave energy is exponential. A small increase in wave height can result in a disproportionately large increase in wave energy.
This is why even seemingly modest waves can pose a significant threat to coastal structures and human safety.
The force exerted by a wave on a coastal structure, such as a seawall or pier, is directly related to its height. Larger waves exert greater pressure, increasing the risk of damage or collapse.
Coastal engineers carefully consider wave height when designing coastal defenses to ensure they can withstand the expected wave forces in a particular location.
Wavelength is the horizontal distance between two successive crests or troughs of a wave. It is typically measured in meters and is a critical factor influencing wave behavior.
In deep water, where the water depth is greater than half the wavelength, waves behave differently than in shallow water, where the depth is less than half the wavelength.
In deep water, waves are relatively unaffected by the seabed. However, as waves enter shallow water, the seabed begins to interact with the wave, slowing it down and causing the wavelength to decrease.
This shortening of the wavelength contributes to an increase in wave height, as the wave's energy is compressed into a smaller volume.
Wavelength dictates wave speed. Longer wavelengths typically mean faster waves, given constant period. As waves approach the shore, the wavelength decreases, leading to changes in wave steepness.
Wavelength is crucial in understanding how waves propagate and interact with coastal features. The longer the wavelength, the more energy a wave typically carries, and the further it can travel without dissipating.
Wave period is the time it takes for two successive crests or troughs to pass a fixed point. It is usually measured in seconds and is inversely related to wave frequency (the number of waves passing a point per unit time).
Wave period can be measured using similar techniques as wave height, including wave buoys and visual observation. Wave buoys record the time between successive crests, providing accurate measurements of wave period.
Visual observation involves timing the passage of several waves and calculating the average period. Wave period is a crucial indicator of wave behavior and the energy being transferred.
Wave period (T) and wave frequency (f) are reciprocals of each other: f = 1/T. A shorter wave period corresponds to a higher wave frequency, and vice versa.
Wave frequency is often used in scientific contexts to describe wave behavior. The relationship between wave period and frequency is fundamental in understanding wave dynamics.
Wave period is critical for predicting wave behavior and its impact on coastal areas. Longer-period waves tend to be more powerful and can travel greater distances, making them a significant factor in coastal erosion and flooding.
Understanding the wave period allows coastal managers to assess the potential risks associated with incoming waves and develop appropriate mitigation strategies.
Ocean waves do not simply travel unchanged from the deep ocean to the coastline. As they approach the shore, they undergo significant transformations that dramatically alter their characteristics and impact.
Understanding these transformations is crucial for predicting wave behavior and mitigating coastal hazards.
Wave Transformations: Shoaling, Interference, and Breaking
As waves venture from the open ocean toward the coastline, they become subject to a series of transformative processes. These changes—shoaling, interference, and ultimately breaking—dictate how wave energy is released and distributed along the shore.
Understanding these transformations is key to predicting their impact and safeguarding coastal communities.
The Shoaling Phenomenon: A Gradual Ascent
Shoaling refers to the changes a wave undergoes as it moves into shallower water. As the water depth decreases, the wave begins to interact with the seabed, and several of its characteristics change.
The most noticeable change is a decrease in wave speed. The wavelength also shortens as the wave's energy becomes compressed.
Increasing Wave Height and Steepness
As a wave shoals, its height increases, and its steepness (the ratio of wave height to wavelength) also increases. This occurs because the wave's energy is being forced into a smaller volume of water.
The increasing steepness makes the wave less stable and more prone to breaking. This transformation is critical in the overall impact of wave energy on the coastline.
Constructive Interference: When Waves Collide
Constructive interference occurs when two or more waves coincide in phase. When their crests and troughs align, their amplitudes add together, resulting in a larger wave.
This phenomenon can lead to the formation of unusually large waves, posing a significant hazard to coastal areas and maritime activities.
Rogue Waves: Unpredictable Giants
One particularly dangerous manifestation of constructive interference is the formation of rogue waves. These are unusually large and unexpected waves that can appear suddenly in the open ocean or near the coast.
Rogue waves are notoriously difficult to predict and can cause significant damage to ships and coastal structures. Their formation mechanisms are still under investigation, but constructive interference is believed to play a significant role.
Breaking Waves: Releasing Energy on the Shoreline
Wave breaking is the final stage in a wave's journey toward the coast. It occurs when the wave becomes too steep to support itself and collapses.
The type of breaking wave depends on the slope of the seabed and the wave's characteristics.
Types of Breaking Waves
There are primarily three types of breaking waves: spilling, plunging, and surging.
-
Spilling breakers occur on gently sloping beaches and are characterized by a gradual spilling of water down the wave's front.
-
Plunging breakers occur on moderately steep beaches and are characterized by a curling crest that plunges down onto the water's surface.
-
Surging breakers occur on steep beaches and are characterized by a wave that surges up the beach face without breaking.
Impact on Coastal Environments
Breaking waves play a critical role in coastal erosion and sediment transport. The energy released by breaking waves can erode cliffs, dislodge sediment, and transport it along the coastline.
The type of breaking wave also influences the distribution of sediment. Plunging breakers, for example, tend to erode the beach face, while spilling breakers tend to deposit sediment on the upper beach.
The dynamics of breaking waves are key factors in the long-term evolution of coastal landscapes. Understanding wave transformations is thus essential for coastal management and hazard mitigation.
Influences on Wave Formation: Wind, Fetch, Refraction, and Diffraction
Ocean waves are not born in isolation. Their creation and behavior are profoundly influenced by a complex interplay of factors, most notably wind, fetch, refraction, and diffraction. Understanding these elements is essential for comprehending wave dynamics and predicting coastal impacts.
Wind and Fetch: The Genesis of Waves
Wind is the primary driving force behind wave generation. As wind blows across the water's surface, it transfers energy to the water, initiating the formation of small ripples. These ripples grow into larger waves as they continue to absorb energy from the wind.
The fetch plays a crucial role in this process. Fetch refers to the distance over which the wind blows in a consistent direction. A longer fetch allows the wind to transfer more energy to the water, resulting in larger and more powerful waves.
The Relationship Between Wind Speed, Fetch, and Wave Height
The relationship between wind speed, fetch, and wave height is direct and significant. Higher wind speeds and longer fetches produce larger waves. This is because stronger winds have more energy to impart to the water, and longer fetches provide more time and space for waves to develop.
Consequently, coastal areas exposed to prevailing winds and long fetches often experience larger and more frequent waves, impacting coastal erosion and wave transformation patterns.
Wave Refraction: Bending Around the Coastline
As waves approach the shore, they often encounter variations in water depth. This leads to a phenomenon known as wave refraction, which involves the bending of waves as they pass over uneven seafloors. Wave refraction is an important indicator when it comes to understanding wave dynamics. It can alter the direction of waves.
The part of the wave in shallower water slows down, while the part in deeper water maintains its speed. This difference in speed causes the wave crest to bend or refract.
The Influence of Underwater Topography
Underwater topography plays a critical role in shaping wave refraction patterns. Waves tend to bend towards areas of shallower water, such as headlands or submerged ridges.
This focusing of wave energy can lead to increased wave heights and erosion in these areas. Conversely, waves tend to diverge in deeper water, resulting in reduced wave heights and erosion in bays.
Wave Diffraction: Spreading Around Obstacles
Wave diffraction occurs when waves encounter an obstacle, such as a breakwater or a harbor entrance. Instead of being completely blocked, the waves bend around the obstacle and spread into the sheltered area.
Diffraction allows wave energy to propagate into areas that would otherwise be calm, influencing wave patterns within harbors and behind coastal structures. This phenomenon can affect sediment transport and navigation.
Diffraction's Impact on Coastal Structures and Harbors
Wave diffraction is a key consideration in the design of coastal structures and harbors. By understanding how waves diffract around these structures, engineers can optimize their placement and configuration to minimize wave disturbance and ensure safe navigation.
For example, breakwaters are often designed to diffract waves in a way that creates a calm area within a harbor, protecting boats and infrastructure from damaging wave action. Understanding wave diffraction ensures the longevity of coastal structures.
Extreme Wave Events and Coastal Hazards: Tsunamis, Rogue Waves, Storm Surges, and More
The ocean, while a source of beauty and sustenance, can also unleash devastating forces upon coastal communities. Understanding the nature and impact of extreme wave events and associated coastal hazards is crucial for effective risk management and mitigation. This section will delve into the characteristics of tsunamis, rogue waves, storm surges, rip currents, coastal erosion, and coastal flooding, shedding light on their causes, impacts, and potential mitigation strategies.
Tsunamis: Walls of Water
Tsunamis are a series of waves caused by large-scale disturbances, most commonly underwater earthquakes. These seismic events displace massive volumes of water, generating waves that radiate outward in all directions. Unlike wind-driven waves, tsunamis have extremely long wavelengths, often exceeding hundreds of kilometers, and can travel at speeds of up to 800 kilometers per hour in the open ocean.
In deep water, tsunamis may be imperceptible, with wave heights of only a few centimeters. However, as they approach the shore, their speed decreases, and their wave height dramatically increases. This phenomenon, known as shoaling, can result in towering walls of water that inundate coastal areas, causing widespread destruction and loss of life.
Devastating Coastal Impacts
The impacts of tsunamis are catastrophic. The sheer force of the water can demolish buildings, infrastructure, and vegetation, sweeping away everything in its path. Coastal communities are particularly vulnerable, as they are often densely populated and lack adequate protection.
The 2004 Indian Ocean tsunami and the 2011 Tohoku tsunami in Japan serve as stark reminders of the destructive power of these natural disasters. These events resulted in immense loss of life, displacement of populations, and long-term economic disruption.
Rogue Waves: Unpredictable Giants
Rogue waves, also known as freak waves or killer waves, are unusually large and unpredictable waves that can appear seemingly out of nowhere. These waves are significantly higher than the surrounding waves and can pose a serious threat to ships and offshore structures.
The formation mechanisms of rogue waves are complex and not fully understood. However, they are often attributed to constructive interference, where multiple wave crests merge to create a single, exceptionally large wave. This can occur due to wave focusing, currents, or nonlinear effects.
A Maritime Hazard
Rogue waves are a major hazard to maritime activities. Their steepness and size can overwhelm even the largest vessels, leading to capsizing or structural damage. The unpredictable nature of rogue waves makes them particularly dangerous, as mariners have little or no warning before encountering them.
Numerous ships have been lost or damaged due to rogue wave encounters. The Draupner wave, recorded in the North Sea in 1995, was one of the first scientifically documented rogue waves and provided valuable insights into their characteristics.
Storm Surges: Coastal Inundation
Storm surges are abnormal rises in sea level during storms, such as hurricanes and cyclones. They are caused by the combined effects of low atmospheric pressure and strong winds pushing water towards the coast. Storm surges can be particularly devastating when they coincide with high tide, resulting in extreme coastal flooding.
The height of a storm surge depends on several factors, including the intensity and size of the storm, the shape of the coastline, and the local bathymetry. Low-lying coastal areas are particularly vulnerable to storm surges.
Amplified Wave Heights and Coastal Flooding
Storm surges amplify wave heights, allowing waves to reach further inland and inundate coastal areas. The combination of high water levels and powerful waves can cause widespread damage to infrastructure, homes, and businesses. Storm surges can also lead to saltwater intrusion into freshwater sources, contaminating drinking water and agricultural lands.
Hurricane Katrina in 2005 and Superstorm Sandy in 2012 are examples of devastating storm surge events that caused widespread flooding and destruction along the US coastline.
Rip Currents: The Silent Threat
Rip currents are strong, narrow currents that flow away from the shore. They are formed when water that has been pushed towards the beach by waves accumulates and then flows back out to sea through a narrow channel. Rip currents can be extremely dangerous, as they can quickly carry swimmers away from the shore.
Rip currents are often difficult to spot, but they can sometimes be identified by a break in the pattern of incoming waves, a channel of churning, choppy water, or a line of foam or debris moving seaward.
Identifying and Escaping Rip Currents
The best way to avoid rip currents is to swim at beaches with lifeguard supervision and to heed any warnings or advisories. If you do get caught in a rip current, it is important to remain calm and not to panic. Do not try to swim directly back to shore against the current. Instead, swim parallel to the shore until you are out of the current, and then swim back to the beach at an angle.
If you are unable to swim out of the current, float or tread water and call for help.
Beach Erosion: A Gradual Loss
Beach erosion is the gradual loss of sand or sediment from a beach. It is a natural process that occurs due to wave action, currents, wind, and sea level rise. However, human activities, such as coastal development and the construction of seawalls, can accelerate beach erosion.
Wave action is a major cause of beach erosion, especially during storms. Large waves can remove significant amounts of sand from the beach, leading to a loss of recreational space, damage to coastal infrastructure, and increased vulnerability to coastal flooding.
The Consequences of Erosion
The consequences of beach erosion can be significant. Loss of beach area can reduce tourism revenue, damage coastal property, and threaten coastal ecosystems. Beach erosion can also expose coastal communities to increased risks from storm surges and coastal flooding.
Coastal Flooding: A Growing Threat
Coastal flooding is the inundation of coastal areas by seawater. It can be caused by a variety of factors, including storm surges, high tides, sea level rise, and land subsidence. Coastal flooding is a growing threat due to climate change, which is causing sea levels to rise and storms to become more intense.
Factors Contributing to Flooding
Sea level rise is a major contributor to coastal flooding. As sea levels rise, coastal areas become more vulnerable to inundation during high tides and storms. Other factors that contribute to coastal flooding include land subsidence, which is the sinking of land due to natural or human-induced causes, and the loss of natural coastal defenses, such as wetlands and mangroves.
Coastal Protection and Management: Strategies for Resilience
Coastal zones are dynamic interfaces where land and sea meet, making them incredibly vulnerable to the relentless forces of nature. Effective coastal management is therefore not merely desirable, but absolutely essential for mitigating hazards, protecting infrastructure, and ensuring the long-term sustainability of these vital regions. This section explores various strategies employed in coastal protection and management, underscoring the critical role of coastal engineers and the impact of structural and non-structural approaches.
The Imperative of Coastal Management
Coastal management is paramount for minimizing the impacts of natural hazards such as erosion, flooding, and storm surges. These hazards can cause significant economic losses, displacement of communities, and damage to critical infrastructure.
Proactive management strategies are necessary to safeguard coastal resources, preserve biodiversity, and maintain the economic viability of coastal regions.
Ignoring the dynamics of coastal environments can lead to irreversible damage and escalating risks in the face of climate change and rising sea levels.
The Role of Coastal Engineers
Coastal engineers are at the forefront of designing and implementing protective measures. Their expertise is crucial in understanding complex coastal processes, assessing risks, and developing sustainable solutions.
These professionals use a blend of scientific knowledge, engineering principles, and innovative technologies to protect coastal communities and infrastructure.
Coastal engineers conduct detailed site investigations, analyze wave patterns and sediment transport, and model the potential impacts of various coastal hazards.
Developing Protective Measures
Based on their assessments, coastal engineers design and implement a range of protection measures, including structural solutions like seawalls and breakwaters, as well as non-structural approaches like beach nourishment and managed retreat.
They work closely with policymakers, environmental scientists, and local communities to develop comprehensive coastal management plans that balance protection with environmental sustainability.
The integration of green infrastructure, such as dune restoration and mangrove planting, is increasingly recognized as a vital component of effective coastal engineering.
Structural Coastal Defenses
Structural coastal defenses are engineered structures designed to physically protect coastlines from wave action and erosion. While effective, their implementation requires careful consideration of potential environmental impacts.
Seawalls
Seawalls are vertical or sloping structures built parallel to the shoreline. They are designed to protect landward areas from wave attack and erosion. Seawalls can be constructed from a variety of materials, including concrete, rock, and steel.
While effective at protecting the immediate area behind them, seawalls can sometimes exacerbate erosion on adjacent beaches by reflecting wave energy.
Proper design and maintenance are critical to ensure the long-term effectiveness of seawalls.
Breakwaters
Breakwaters are offshore structures designed to reduce wave energy reaching the shoreline. They create a sheltered area behind them, providing protection for harbors, beaches, and coastal infrastructure.
Breakwaters can be either attached to the shore or detached, depending on the specific site conditions and protection goals.
The design of breakwaters must consider factors such as wave climate, sediment transport, and potential impacts on marine ecosystems.
Non-Structural Approaches: Soft Engineering
Non-structural approaches, often referred to as “soft engineering,” focus on working with natural processes to protect coastlines. These methods are generally more environmentally friendly and sustainable than structural solutions.
Beach Nourishment
Beach nourishment involves adding sand to an eroding beach to widen it and provide a buffer against wave action. This can be achieved by dredging sand from offshore or transporting it from inland sources.
Beach nourishment provides recreational benefits and enhances the natural appearance of the coastline.
However, it is a recurring process, as the added sand will eventually be eroded by waves and currents.
Managed Retreat
Managed retreat involves the strategic relocation of infrastructure and development away from vulnerable coastal areas. This approach recognizes that in some cases, it is more sustainable to retreat from the coast than to fight against natural forces.
Managed retreat can be a controversial strategy, as it may require landowners to give up their property.
However, it can be a cost-effective and environmentally sound solution in areas where erosion rates are high and structural defenses are not feasible.
Balancing Protection and Preservation
Effective coastal management requires a balanced approach that considers both protection and preservation.
Integrating structural and non-structural measures, along with sustainable development practices, is essential for ensuring the long-term resilience of coastal communities.
Continued research, monitoring, and adaptive management are crucial for responding to the evolving challenges of coastal erosion, sea level rise, and climate change.
Monitoring and Prediction: Tools for Understanding Wave Behavior
The ability to accurately monitor and predict wave behavior is paramount for coastal safety, navigation, and resource management. A suite of sophisticated tools and technologies are employed to achieve this, providing invaluable data and insights into the complex dynamics of ocean waves.
These tools range from in-situ measurements collected by wave buoys to the expansive views provided by satellite imagery and the predictive power of numerical wave models.
The Role of Wave Buoys
Wave buoys are indispensable instruments for directly measuring wave characteristics at specific locations. Positioned strategically across the world's oceans, these floating platforms continuously collect and transmit data on wave height, wave period, and wave direction.
The data obtained from wave buoys are crucial for validating wave models, providing real-time information for mariners, and tracking long-term trends in wave climate.
Wave Height Measurement
Wave buoys typically utilize accelerometers or GPS technology to measure the vertical displacement of the buoy caused by passing waves.
This data is then processed to determine significant wave height, which represents the average height of the highest one-third of waves in a given period. This metric is particularly useful for assessing the potential impact of waves on coastal structures and vessels.
Wave Period and Direction
In addition to wave height, wave buoys also measure the time between successive wave crests, known as the wave period.
Furthermore, they can determine the direction from which waves are approaching, providing valuable information for understanding wave propagation patterns.
This is often achieved using compasses or by analyzing the buoy's motion in three dimensions.
Satellite Imagery: A Broad View
Satellite imagery offers a unique perspective on ocean wave dynamics, providing a synoptic view of wave patterns across vast areas.
Satellites equipped with synthetic aperture radar (SAR) can measure wave height and direction by analyzing the backscatter of radar signals from the ocean surface.
This data is particularly useful for identifying large-scale wave features, such as swell patterns and storm waves, that may not be captured by individual wave buoys.
Applications of Satellite Data
Satellite imagery also plays a critical role in monitoring sea ice, detecting oil spills, and tracking harmful algal blooms, all of which can indirectly influence wave behavior and coastal safety.
The combination of satellite data with other sources of information provides a comprehensive understanding of ocean conditions.
Numerical Wave Models: Simulating the Ocean
Numerical wave models are sophisticated computer programs that simulate the generation, propagation, and dissipation of ocean waves.
These models use mathematical equations to represent the physical processes that govern wave behavior, taking into account factors such as wind speed, water depth, and bottom topography.
How Numerical Models Work
By inputting real-time and historical data on these factors, wave models can predict future wave conditions with remarkable accuracy. These models are essential for forecasting coastal flooding, planning maritime operations, and designing coastal defenses.
Several advanced numerical models are used globally to predict wave behavior. Some popular models include WAVEWATCH III and SWAN (Simulating Waves Nearshore).
Model Limitations and Advancements
Despite their capabilities, numerical wave models have limitations. Their accuracy depends on the quality and resolution of the input data, as well as the complexity of the model itself.
Ongoing research and development efforts are focused on improving the accuracy and efficiency of wave models.
Scientists accomplish this by incorporating new data sources, refining the underlying equations, and leveraging the power of high-performance computing.
Video: Crest of a Wave: Ocean Dynamics & Coastal Safety
FAQs: Crest of a Wave - Ocean Dynamics & Coastal Safety
What's meant by "ocean dynamics"?
Ocean dynamics refers to the forces and processes that drive ocean movement. This includes waves, tides, currents, and the interactions between them. Understanding these dynamics is crucial for predicting where the "crest of a wave" will break and how coastlines are shaped.
Why is understanding coastal safety important?
Coastal safety is vital because coastal areas are subject to powerful natural forces. Knowledge of rip currents, wave heights, and tide changes helps people make informed decisions. Ignoring these factors can put you at risk when a "crest of a wave" is forming.
How do tides affect coastal conditions?
Tides significantly influence water levels, impacting wave heights and currents. High tide can lead to larger waves and increased flooding, while low tide can expose hazardous areas. The changing tide affects when and where you might see the "crest of a wave" become a threat.
What are rip currents and how do I avoid them?
Rip currents are strong, narrow currents that flow away from the shore. They are dangerous because they can quickly pull swimmers out to sea. To avoid them, swim at guarded beaches and learn to identify them. If caught in one, swim parallel to the shore until you're out of the current, then swim to safety, avoiding being pulled under the "crest of a wave."
So, next time you're at the beach, remember that the seemingly simple crest of a wave is just the tip of the iceberg when it comes to understanding the ocean's power. Stay informed, stay aware, and most importantly, stay safe out there!