Condensation Point of Oxygen: Guide & FAQs
Oxygen, a critical element for biological respiration and combustion, transitions from its gaseous state to a liquid state at a specific temperature. This temperature, known as the condensation point of oxygen, is quantitatively defined by thermodynamics and is heavily relied upon in various industrial applications. Cryogenics, the branch of physics that studies the production and effects of very low temperatures, provides essential methodologies for achieving these extremely low temperatures required to observe condensation. The Linde Group, a leading industrial gas company, employs oxygen condensation extensively in air separation processes to produce pure oxygen for medical, industrial, and research purposes. Precise measurement of the condensation point of oxygen requires specialized instruments and techniques similar to those developed by Heike Kamerlingh Onnes, a pioneer in the field of cryogenics and Nobel laureate for his work on the properties of matter at low temperatures.
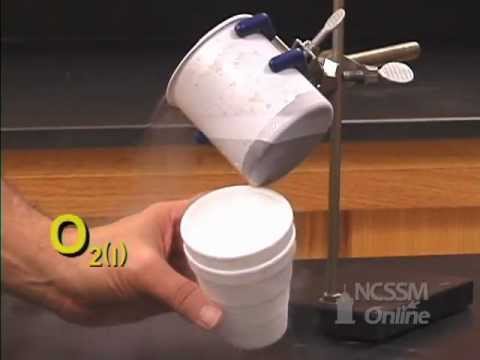
Image taken from the YouTube channel North Carolina School of Science and Mathematics , from the video titled Condensing O2 .
Unveiling the Mysteries of Oxygen Condensation
Condensation, a ubiquitous phenomenon in both the natural world and industrial processes, plays a crucial role in numerous aspects of our lives. From the formation of morning dew on grass to the sophisticated processes within cryogenic engineering, understanding condensation is paramount.
Defining Condensation: From Gas to Liquid
At its core, condensation is the phase transition of a substance from its gaseous state to its liquid state. This occurs when the gas is cooled to its condensation point or subjected to increased pressure. During condensation, gas molecules lose kinetic energy, causing them to slow down and allowing intermolecular forces to draw them closer together. This process results in the formation of a liquid.
The Significance of Condensation in Science and Everyday Life
Condensation is not merely a laboratory curiosity. It is a fundamental process driving weather patterns, such as cloud formation and precipitation. It is also essential in various industrial applications, including distillation, refrigeration, and power generation. Understanding and controlling condensation is vital for optimizing these processes and ensuring their efficiency.
The Critical Importance of Oxygen's Condensation Point
The condensation point of oxygen is of particular interest due to its wide-ranging applications across diverse sectors. From the life-saving applications in medical oxygen therapy to its critical role as an oxidizer in rocket propulsion, liquid oxygen (LOX) is indispensable. A thorough understanding of the specific conditions required to condense oxygen, and the properties of LOX, is crucial for safe and effective utilization.
Scope of This Exploration: A Comprehensive Guide
This exploration will delve into the fascinating world of oxygen condensation. We will begin by examining the fundamental scientific principles that govern condensation in general, including phase transitions, intermolecular forces, and vapor pressure.
We will then focus specifically on oxygen, exploring its unique properties and the factors that influence its condensation point.
Next, the practical applications of liquid oxygen across various industries will be examined. The exploration will also extend to the equipment and technologies employed to achieve oxygen condensation and safely handle liquid oxygen.
Finally, we will address frequently asked questions regarding oxygen condensation. This comprehensive overview aims to provide a thorough understanding of this essential scientific and industrial process.
The Science Behind Condensation: A Deep Dive
To fully grasp the intricacies of oxygen condensation, it is essential to first understand the fundamental scientific principles that govern this fascinating phenomenon. This section will delve into the core concepts underpinning condensation, providing a detailed exploration of phase transitions, intermolecular forces, vapor pressure, and the thermodynamic principles at play.
Understanding Phase Transitions
Matter exists in various states, most commonly solid, liquid, and gas. These states are referred to as phases, and the transition from one phase to another is known as a phase transition. Examples of phase transitions include melting (solid to liquid), boiling (liquid to gas), freezing (liquid to solid), and condensation (gas to liquid).
The phase of a substance depends primarily on temperature and pressure. For instance, water exists as ice (solid) at low temperatures, liquid water at moderate temperatures, and steam (gas) at high temperatures.
Liquefaction: Converting Gas to Liquid
Liquefaction is the process of converting a substance from its gaseous state into a liquid state. This is achieved by either cooling the gas below its critical temperature or by increasing the pressure on the gas, or both. Liquefaction is a crucial step in the condensation of oxygen.
During liquefaction, the kinetic energy of gas molecules decreases, causing them to slow down and come closer together. This allows intermolecular forces to become dominant, leading to the formation of a liquid.
The Role of Intermolecular Forces
Intermolecular forces are the attractive or repulsive forces that exist between molecules. These forces play a critical role in determining the physical properties of a substance, including its boiling point and condensation point.
In the gaseous state, molecules have high kinetic energy and are widely separated, with intermolecular forces being relatively weak. However, as the gas is cooled, the kinetic energy decreases, allowing intermolecular forces to draw the molecules closer together, eventually leading to condensation.
The strength of intermolecular forces varies depending on the type of molecule. Stronger intermolecular forces result in higher boiling and condensation points.
Vapor Pressure and Condensation Point
Vapor pressure is the pressure exerted by a vapor in thermodynamic equilibrium with its condensed phases (solid or liquid) at a given temperature in a closed system. The condensation point of a substance is the temperature at which the vapor pressure of the liquid equals the surrounding atmospheric pressure.
When the temperature of a gas decreases to the point where its vapor pressure equals the surrounding pressure, condensation begins to occur. The lower the vapor pressure at a given temperature, the easier it is for the substance to condense.
The Effect of Pressure on Condensation
Pressure plays a significant role in determining the condensation point of a substance. Generally, increasing the pressure on a gas raises its condensation point. This is because increased pressure forces the gas molecules closer together, enhancing intermolecular forces and facilitating the transition to the liquid phase.
Conversely, decreasing the pressure lowers the condensation point. This principle is utilized in various industrial processes involving the liquefaction and separation of gases.
Boiling Point and Condensation Point: A Close Relationship
The boiling point and condensation point of a substance are essentially the same temperature at a given pressure. The boiling point is the temperature at which a liquid changes to a gas, while the condensation point is the temperature at which a gas changes to a liquid.
This equivalence is due to the fact that both processes involve the same energy changes related to overcoming intermolecular forces.
Latent Heat: Energy Released During Condensation
Latent heat is the heat absorbed or released during a phase transition at a constant temperature. During condensation, heat is released as the gas molecules transition to the liquid phase. This heat is known as the latent heat of condensation.
The release of latent heat during condensation is a crucial factor in various natural and industrial processes, affecting temperature regulation and energy transfer.
Thermodynamics of Phase Changes
Thermodynamic principles govern phase changes, providing a framework for understanding the energy and entropy changes involved. Enthalpy (H) is a thermodynamic property that represents the total heat content of a system. Entropy (S) is a measure of the disorder or randomness of a system.
During condensation, the enthalpy of the system decreases as heat is released. The entropy also decreases as the gas molecules become more ordered in the liquid phase. These changes are governed by the laws of thermodynamics, which dictate the direction and spontaneity of phase transitions.
Cryogenics is the science that deals with the production and effects of very low temperatures. It is particularly relevant to oxygen condensation, as oxygen must be cooled to extremely low temperatures to transition into its liquid state.
Cryogenic techniques are used to achieve the necessary temperatures for liquefying oxygen and other gases, enabling a wide range of applications in fields such as medicine, aerospace, and industrial gas production.
Oxygen: Properties, Behavior, and its Unique Condensation Point
Oxygen, the life-sustaining element that comprises approximately 21% of Earth's atmosphere, exhibits a range of properties and behaviors that are critical to understanding its condensation point. This section will delve into the specific characteristics of oxygen, both in its gaseous and liquid states, and explore the factors that influence its phase transition.
Chemical and Physical Properties of Oxygen (O2)
Oxygen, with the chemical formula O2, is a diatomic molecule at standard temperature and pressure. It is a colorless, odorless, and tasteless gas.
Oxygen is paramagnetic, meaning it is weakly attracted to magnetic fields due to the presence of unpaired electrons. This property distinguishes it from many other common gases.
Oxygen is a highly reactive element, readily forming oxides with most other elements. This reactivity is fundamental to processes like combustion and corrosion.
Its molecular weight is approximately 32 g/mol. It has a density of around 1.429 g/L at standard temperature and pressure.
Unique Properties of Liquid Oxygen (LOX)
When cooled below its condensation point, oxygen transitions into its liquid state, commonly referred to as LOX. Liquid oxygen possesses several unique properties that are distinct from its gaseous form.
LOX has a pale blue color, which arises from the absorption of red light.
The density of LOX is significantly higher than that of gaseous oxygen, approximately 1.141 g/mL. This high density makes LOX an efficient storage medium for oxygen.
LOX is also a powerful oxidizer. Contact with organic materials can result in rapid and intense combustion.
Due to its extremely low temperature, LOX can cause severe cryogenic burns upon contact with skin.
Oxygen vs. Nitrogen: A Comparative Analysis
Nitrogen, another major component of air, shares some similarities with oxygen but also exhibits key differences, especially concerning its condensation point.
Nitrogen's condensation point is lower than that of oxygen.
Under standard conditions, oxygen condenses at approximately -183°C (90.2 K), while nitrogen condenses at approximately -196°C (77.3 K). This difference in condensation points is exploited in the industrial separation of oxygen and nitrogen from air.
Nitrogen is relatively inert compared to oxygen. It does not readily participate in combustion.
The different electronic structures and intermolecular forces between oxygen and nitrogen contribute to their varying condensation points.
Condensation Point of Oxygen Under Standard Conditions
The condensation point of oxygen (O2) under standard atmospheric pressure (1 atm) is -183°C (90.2 K or -297.4°F).
This is the temperature at which gaseous oxygen will transition into liquid oxygen when cooled at standard pressure.
This value is a fundamental reference point in cryogenics and related fields. It is crucial for designing systems that utilize or process liquid oxygen.
Factors Influencing the Condensation Point of Oxygen
Several factors can influence the precise condensation point of oxygen. The two most prominent are pressure and purity.
Pressure
As discussed earlier, increasing the pressure on a gas generally raises its condensation point. Conversely, decreasing the pressure lowers the condensation point.
This relationship is described by the Clausius-Clapeyron equation, which mathematically relates vapor pressure and temperature.
Purity
The presence of impurities in oxygen can also affect its condensation point.
Impurities can disrupt the intermolecular forces between oxygen molecules. This leads to a change in the energy required for phase transition.
Even small amounts of contaminants can measurably alter the condensation temperature. For example, other gases like argon or nitrogen, if mixed with oxygen, could shift the condensation temperature.
Liquid Oxygen in Action: Practical Applications Across Industries
Liquid oxygen (LOX), owing to its unique properties and exceptionally low temperature, finds widespread application across a multitude of industries. Its role extends far beyond the laboratory, permeating critical sectors such as cryogenics, aerospace, medicine, and industrial gas production. The versatility of LOX stems from its high oxygen concentration and its ability to support or enable processes impossible at ambient temperatures.
Cryogenics: Enabling Extreme Low-Temperature Applications
The cryogenics industry hinges on the manipulation of materials at extremely low temperatures, typically below -150°C (123 K). LOX plays a pivotal role in this field, serving as a coolant or a reactive agent in various applications.
A prominent example is its use in magnetic resonance imaging (MRI) machines. Superconducting magnets within MRI systems require continuous cooling to achieve and maintain superconductivity. LOX, or more commonly liquid helium, is employed to cool these magnets, ensuring optimal imaging performance.
Other cryogenic applications involving LOX include:
- Cryopreservation: Preserving biological samples (cells, tissues, organs) at ultra-low temperatures for extended periods.
- Superconductivity Research: Investigating and developing new superconducting materials that exhibit zero electrical resistance at cryogenic temperatures.
- Cryoelectronics: Developing electronic devices that operate more efficiently at cryogenic temperatures.
Aerospace: Powering Rocket Propulsion
In the aerospace industry, LOX is an indispensable component of rocket propulsion systems. It serves as a powerful oxidizer, reacting with a fuel (typically kerosene or liquid hydrogen) to generate thrust.
The high energy density of LOX, coupled with its relatively low molecular weight, makes it an ideal oxidizer for rocket engines. The combustion of LOX and fuel produces a large volume of hot gas, which is then expelled through a nozzle to generate thrust.
The Space Shuttle Main Engines (SSMEs), for example, utilized LOX as an oxidizer in conjunction with liquid hydrogen as fuel, achieving remarkable performance and reliability. Modern rocket designs continue to rely on LOX as a key component of their propulsion systems, including those used by SpaceX, Blue Origin, and NASA.
Medical Field: Supporting Life and Enhancing Treatment
LOX plays a crucial role in the medical field, primarily in breathing apparatus and oxygen therapy. Patients with respiratory ailments, such as chronic obstructive pulmonary disease (COPD) or pneumonia, often require supplemental oxygen to maintain adequate blood oxygen levels.
LOX is stored in portable or stationary containers and delivered to patients through masks or nasal cannulas. This provides a concentrated source of oxygen, improving breathing and overall health.
Beyond respiratory support, LOX is also used in:
- Hyperbaric oxygen therapy: Enhancing the oxygen supply to tissues for wound healing and treating certain medical conditions.
- Cryosurgery: Freezing and destroying diseased tissues, such as tumors or warts.
- Medical research: Studying the effects of oxygen on various biological processes.
Industrial Gas Production: A Foundation for Many Industries
The industrial gas production sector is responsible for manufacturing and distributing various gases, including oxygen, nitrogen, argon, and carbon dioxide. LOX serves as a key intermediate in the production of gaseous oxygen and is also sold directly for various industrial applications.
The primary method for producing LOX is cryogenic air separation. This process involves cooling air to extremely low temperatures, causing the various components (nitrogen, oxygen, argon) to condense at different temperatures.
These components are then separated through distillation, resulting in high-purity LOX. Key companies in the industrial gas production field, such as Linde, Air Liquide, and Air Products, operate large-scale air separation plants to produce LOX and other industrial gases. This LOX is then used in steelmaking, welding, chemical processing, and countless other industries.
Tools of the Trade: Equipment and Technologies for Oxygen Condensation
The condensation of oxygen, a process fundamental to various industries, relies on sophisticated equipment and technologies. These tools are engineered to achieve and maintain the extremely low temperatures required for oxygen to transition from a gaseous to a liquid state. From specialized cooling systems to secure storage solutions, each component plays a critical role in the safe and efficient handling of liquid oxygen (LOX).
Cryogenic Coolers: Achieving Ultra-Low Temperatures
Cryogenic coolers are the workhorses of oxygen condensation, responsible for generating the frigid conditions necessary to liquefy the gas. Several technologies are employed in these coolers, each with its strengths and limitations.
Joule-Thomson Effect
The Joule-Thomson effect, also known as the Joule-Kelvin effect, is a thermodynamic process where the temperature of a real gas changes when it is forced through a valve or porous plug while keeping it insulated from its environment. This isenthalpic process can lead to cooling, especially when the gas is below its inversion temperature. This principle is leveraged in some cryogenic coolers to pre-cool gases before further liquefaction.
Pulse Tube Refrigerators
Pulse tube refrigerators (PTRs) are another important cooling technology. They offer high reliability and efficiency and are commonly used in laboratory settings and industrial applications. PTRs use pressure waves generated by a compressor to create a temperature gradient within a tube, resulting in cooling.
Gifford-McMahon (GM) Refrigerators
Gifford-McMahon (GM) refrigerators are mechanically driven systems that use a displacer and a regenerator to achieve cryogenic temperatures. They are widely used due to their relatively simple design and ability to reach temperatures down to around 10 K (-263 °C). GM refrigerators are often found in applications such as cooling superconducting magnets and cryopumps.
Distillation Columns: Separating Oxygen from Air
Air is a mixture of gases, primarily nitrogen and oxygen. To obtain pure liquid oxygen, air separation techniques are employed, with distillation columns playing a central role. The process leverages the difference in boiling points between nitrogen (-196 °C) and oxygen (-183 °C).
In a cryogenic air separation unit (ASU), air is first compressed, purified to remove contaminants like water and carbon dioxide, and then cooled to cryogenic temperatures. The cooled air enters the distillation column, where it is separated into liquid nitrogen and liquid oxygen.
The distillation column typically consists of multiple stages or trays. As the liquid mixture flows down the column, it comes into contact with vapor rising up. The more volatile nitrogen vaporizes and rises to the top of the column, while the less volatile oxygen condenses and flows to the bottom. This continuous process results in highly pure liquid oxygen.
Liquid Oxygen Tanks: Design and Safety Considerations
Liquid oxygen must be stored in specialized tanks designed to withstand the extremely low temperatures and high pressures associated with the liquid. Safety is paramount in the design and operation of these tanks.
Insulation
Effective insulation is crucial to minimize heat leak into the tank, which would cause the LOX to vaporize. Vacuum insulation, often combined with multilayer insulation (MLI), is commonly used. MLI consists of multiple layers of thin, reflective material separated by a vacuum, effectively reducing heat transfer by radiation and conduction.
Pressure Relief Valves
Pressure relief valves are essential safety devices that prevent over-pressurization of the tank. As LOX warms and vaporizes, the pressure inside the tank increases. If the pressure exceeds a safe limit, the relief valve automatically opens, releasing excess pressure and preventing a potentially catastrophic rupture.
Material Selection
The materials used in the construction of LOX tanks must be compatible with cryogenic temperatures. Stainless steel and aluminum alloys are commonly used due to their good strength and ductility at low temperatures. These materials are also resistant to corrosion and do not become brittle at cryogenic temperatures.
Pressure Gauges: Monitoring System Pressure
Accurate pressure monitoring is crucial for the safe and efficient operation of LOX systems. Pressure gauges provide real-time information about the pressure inside the tanks and pipelines. These gauges must be calibrated and maintained regularly to ensure accurate readings. They also provide valuable insight into the health and functionality of the system.
Thermometers: Measuring Cryogenic Temperatures
Precise temperature measurement is essential for controlling the oxygen condensation process and ensuring the stability of LOX storage. Thermometers specifically designed for cryogenic temperatures are used to monitor the temperature of LOX, cooling systems, and related equipment.
Resistance temperature detectors (RTDs) and thermocouples are common types of cryogenic thermometers. RTDs measure temperature based on the change in electrical resistance of a metal wire, while thermocouples measure temperature based on the thermoelectric effect, which generates a voltage proportional to the temperature difference between two dissimilar metals. These thermometers provide critical data for optimizing performance and ensuring safe operation.
Maintaining Standards: Regulatory Agencies and Data Sources
The pursuit of precise knowledge regarding the condensation point of oxygen and its related properties is not merely an academic exercise. It is a foundational requirement for the safe and efficient operation of numerous industries. Ensuring accuracy and consistency in this realm necessitates the rigorous oversight and standardization provided by regulatory agencies and reliable data sources.
The Indispensable Role of NIST
Among these entities, the National Institute of Standards and Technology (NIST) stands as a cornerstone. NIST's crucial role is to furnish authoritative data and develop standards pertaining to the thermodynamic and physical properties of oxygen, including, of course, its condensation point and density across a range of conditions.
This data is not simply collected and disseminated; it is meticulously validated and refined through ongoing research and experimentation. NIST's efforts extend to developing Standard Reference Materials (SRMs), certified substances with precisely known properties, that serve as benchmarks for calibrating instruments and validating analytical methods.
NIST's Databases and Publications
NIST provides researchers, engineers, and industries with access to a wealth of data through its various databases and publications. The NIST Chemistry WebBook, for instance, is an invaluable resource for thermochemical data, including the properties of oxygen at different temperatures and pressures.
Furthermore, NIST publishes technical reports and peer-reviewed papers detailing its research findings and methodologies. These publications provide the scientific basis for the agency's standards and recommendations. They offer critical insights into the uncertainties associated with various measurements.
How Industries and Researchers Leverage NIST Data
The accuracy and reliability of NIST data are paramount for industries that rely on the condensation of oxygen. The aerospace sector, for example, utilizes liquid oxygen as an oxidizer in rocket propulsion.
Accurate knowledge of oxygen's density and thermodynamic properties is crucial for calculating propellant requirements, designing combustion chambers, and ensuring the safe and efficient operation of rocket engines. Any deviation from the predicted behavior of LOX can have catastrophic consequences.
The medical field also relies heavily on NIST data. In oxygen therapy, the precise delivery of oxygen is critical for patient care. NIST's data enables manufacturers of medical oxygen equipment to accurately calibrate their devices and ensure that patients receive the correct dosage.
Furthermore, the industrial gas sector relies on NIST's data for the design and operation of air separation units (ASUs). These facilities use distillation to separate oxygen from air, and precise knowledge of oxygen's vapor pressure and other thermodynamic properties is essential for optimizing the separation process and maximizing efficiency.
The Importance of Traceability and Calibration
Traceability to NIST standards is a critical requirement for many industries. Calibration of instruments used to measure the properties of oxygen must be traceable to NIST SRMs to ensure accuracy and consistency.
This traceability provides confidence that measurements made in different laboratories and at different times are comparable and reliable. This is essential for ensuring product quality, regulatory compliance, and the safety of operations involving liquid oxygen.
In conclusion, the condensation point of oxygen, though seemingly a single data point, is underpinned by a complex web of scientific inquiry, rigorous standardization, and meticulous data management. Agencies like NIST play an indispensable role in maintaining the accuracy and reliability of this knowledge. This ensures the safety, efficiency, and innovation of industries that rely on oxygen condensation.
Frequently Asked Questions (FAQs) About Oxygen Condensation
This section addresses common queries and misconceptions surrounding the condensation of oxygen, aiming to provide precise and accessible explanations that enhance understanding and dispel potential confusion. Understanding the fundamental properties and safe handling procedures of liquid oxygen (LOX) is crucial, and these FAQs serve as a practical guide.
Core Properties and Handling
Why is Liquid Oxygen (LOX) So Cold?
Liquid oxygen exists at extremely low temperatures, approximately -183°C (-297°F) at standard atmospheric pressure. This is because condensation, the phase transition from gas to liquid, requires the removal of kinetic energy from the oxygen molecules.
To achieve this state, the molecules must be sufficiently slowed down to allow intermolecular forces to dominate, drawing them closer together to form the liquid phase. This dramatic reduction in molecular motion is directly reflected in the low temperature.
What are the Primary Hazards of Working with LOX?
Working with liquid oxygen presents several significant hazards that must be carefully mitigated.
Cryogenic burns are a primary concern, as direct contact with LOX can cause rapid freezing of skin and tissues. Due to its extremely cold nature, it will quickly freeze any exposed tissue.
Oxygen enrichment is another major hazard. LOX can cause materials to become highly flammable or even explosive. Materials that are normally difficult to ignite can burn vigorously or explode in an oxygen-enriched environment.
Asphyxiation can occur if LOX evaporates in a confined space, displacing oxygen and creating an oxygen-deficient atmosphere.
How is Liquid Oxygen Typically Stored?
LOX is typically stored in specialized cryogenic tanks designed to maintain its extremely low temperature and prevent evaporation.
These tanks feature double-walled construction with a vacuum between the walls to provide excellent thermal insulation. Insulation minimizes heat transfer from the surrounding environment, reducing the rate of LOX evaporation.
Pressure relief valves are also crucial components, preventing over-pressurization due to any unavoidable evaporation.
Safety Considerations
What Materials are Compatible with LOX?
Not all materials are suitable for use in contact with liquid oxygen due to the risk of ignition or degradation.
Stainless steel, copper, and certain polymers are commonly used due to their compatibility and resistance to degradation at cryogenic temperatures. These materials generally exhibit good resistance to ignition in oxygen-enriched environments.
Materials such as oil, grease, and many organic compounds are strictly prohibited, as they can react violently with LOX, leading to fires or explosions.
Can LOX be Stored Indefinitely?
While cryogenic tanks are designed to minimize evaporation, LOX cannot be stored indefinitely without losses.
Even with excellent insulation, some heat inevitably leaks into the tank, causing the LOX to slowly evaporate. This evaporation rate, known as the boil-off rate, varies depending on the tank design and ambient conditions.
Regular monitoring and replenishment are necessary to maintain the desired LOX level.
What Safety Precautions Should Be Taken When Handling LOX?
Strict adherence to safety protocols is paramount when handling liquid oxygen.
Personal Protective Equipment (PPE) is essential, including cryogenic gloves, face shields, and appropriate clothing to protect against cryogenic burns.
Adequate ventilation is crucial to prevent oxygen enrichment and asphyxiation hazards.
Regular equipment inspections are also necessary to identify and address any potential leaks or malfunctions.
Training on proper handling procedures and emergency response is indispensable for all personnel working with LOX.
Practical Applications
What Industries Commonly Use Liquid Oxygen?
Liquid oxygen finds extensive use across a wide range of industries.
The aerospace industry relies on LOX as an oxidizer in rocket propulsion systems, providing the necessary oxygen for combustion.
In the medical field, LOX is used for oxygen therapy, providing supplemental oxygen to patients with respiratory conditions.
Industrial gas suppliers produce and distribute LOX for various applications, including welding, cutting, and chemical processing.
Cryogenics applications are also common, taking advantage of LOX's extremely low temperature for various processes.
How is LOX Produced on an Industrial Scale?
The primary method for producing liquid oxygen on an industrial scale is through cryogenic air separation.
This process involves cooling air to extremely low temperatures, causing it to liquefy. The liquid air is then separated into its components, primarily oxygen and nitrogen, based on their different boiling points using distillation columns.
The separated oxygen is then collected and stored as liquid oxygen.
By addressing these frequently asked questions, we aim to provide a clearer understanding of liquid oxygen's properties, hazards, and applications, promoting safer handling and more informed use across various industries.
Video: Condensation Point of Oxygen: Guide & FAQs
FAQs: Condensation Point of Oxygen
What does it mean for oxygen to condense?
Condensation is when a gas changes into a liquid. For oxygen, it means the gaseous oxygen molecules slow down enough that intermolecular forces cause them to clump together and form liquid oxygen. This occurs at the condensation point of oxygen.
What is the condensation point of oxygen in Celsius and Fahrenheit?
The condensation point of oxygen is approximately -183 degrees Celsius (or -297.4 degrees Fahrenheit). At this temperature, oxygen transitions from a gas to a liquid.
Is the condensation point the same as the boiling point?
Yes, the condensation point and the boiling point are the same temperature. The difference is the direction of the phase change. Condensation is gas to liquid, while boiling is liquid to gas, but both occur at the same temperature for a given substance, including the condensation point of oxygen.
Why is knowing the condensation point of oxygen important?
Understanding the condensation point of oxygen is crucial in many fields. It's vital for cryogenic research, industrial applications like steelmaking where liquid oxygen is used, and for safely handling and storing oxygen in its liquid form. It helps manage conditions where oxygen might unexpectedly condense.
So, there you have it! Everything you need to know about the condensation point of oxygen. Hopefully, this guide has cleared up any confusion and answered your burning questions. Now you can confidently discuss oxygen's liquefaction with your friends (or maybe just impress them at the next trivia night!).