Cobalt Electron Configuration: Guide for Students
The electronic structure of cobalt, a crucial element in materials science, determines its chemical behavior and physical properties. Understanding the cobalt electron configuration involves applying the Aufbau principle, which dictates the filling of atomic orbitals in a specific sequence. Linus Pauling’s contributions to the understanding of chemical bonding are particularly relevant when examining how cobalt interacts with other elements based on its electron arrangement. The periodic table, developed by Dmitri Mendeleev, organizes elements like cobalt by their atomic number and electron configurations, providing a framework for predicting cobalt’s behavior in various chemical reactions and magnetic applications.
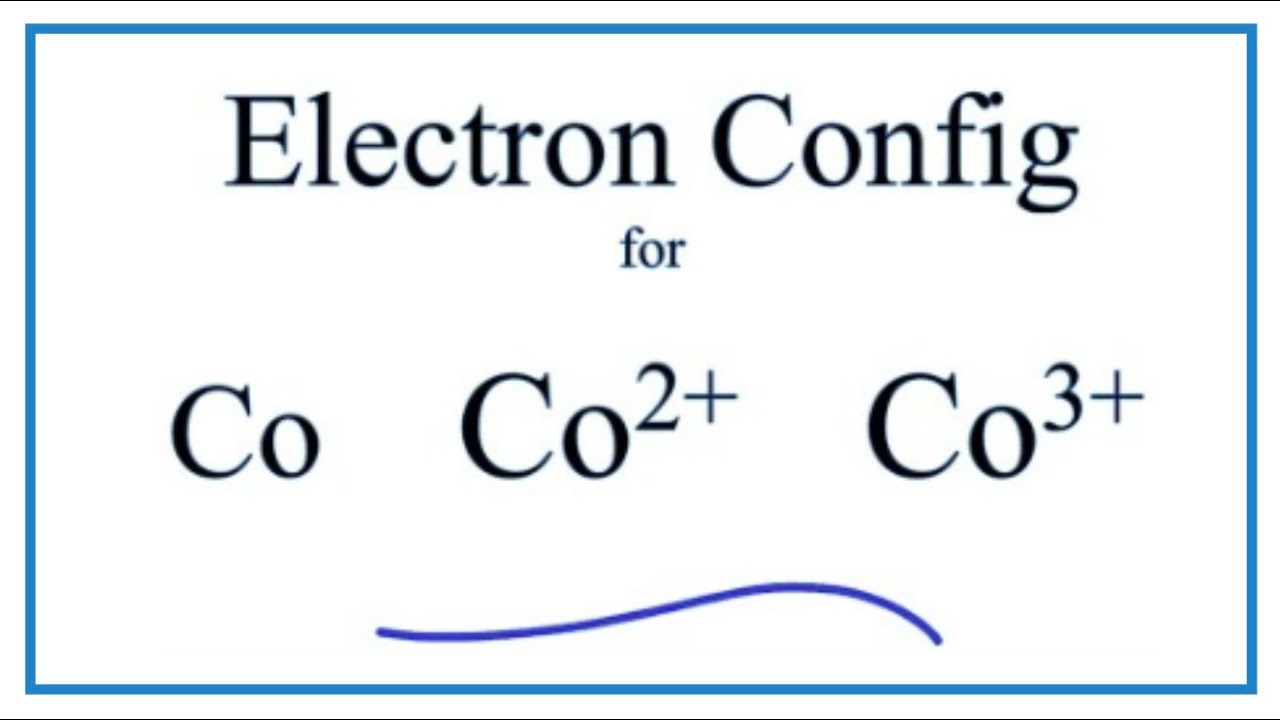
Image taken from the YouTube channel Wayne Breslyn (Dr. B.) , from the video titled Electron Configuration for Co, Co2+, and Co3+ (Cobalt and Cobalt Ions) .
Cobalt: Unveiling the Secrets of its Electron Configuration
Cobalt (Co), a chemical element of significant industrial and biological importance, commands our attention. Understanding its properties necessitates a thorough examination of its electron configuration. This initial exploration sets the stage for a deeper dive into the intricacies of electron arrangement and its profound influence on cobalt's behavior.
Cobalt: An Overview
Cobalt, represented by the symbol Co, is a hard, ductile, and ferromagnetic metal. Its significance stems from its diverse applications. These applications range from high-performance alloys used in jet engines to pigments that impart a vibrant blue hue to glass and ceramics. Cobalt is also a crucial component of vitamin B12, highlighting its biological importance.
Position in the Periodic Table
Cobalt resides in Group 9 (formerly VIIIB) and Period 4 of the periodic table. Its placement amongst the transition metals dictates its characteristic variable oxidation states and its ability to form coordination compounds. This location also signifies that its d orbitals are in the process of being filled, a key factor in determining its electron configuration and properties.
The Role of Electron Configuration
The electron configuration of an element dictates its chemical behavior. It determines how an atom will interact with other atoms to form chemical bonds. The arrangement of electrons within an atom's orbitals dictates its reactivity, ionization energy, and electronegativity. The specific electron configuration is not merely a theoretical construct; it’s the blueprint for understanding the element's behavior.
Atomic Structure and Electron Arrangement
The electron configuration is intimately linked to the atom’s structure. The nucleus, containing protons and neutrons, is surrounded by electrons arranged in specific energy levels or shells. Each shell consists of one or more subshells known as orbitals (s, p, d, and f). These orbitals are defined by specific shapes and energy levels. The precise manner in which electrons populate these orbitals determines the electron configuration, which in turn governs cobalt's chemical characteristics.
Fundamental Concepts: Building Blocks of Electron Arrangement
To comprehend the electron configuration of cobalt, a firm grasp of the underlying principles is essential. These fundamental concepts provide the framework for understanding how electrons are arranged within an atom, dictating its chemical behavior and reactivity. Let us delve into these crucial building blocks.
Atomic Number and Its Significance for Cobalt
The atomic number (Z) is the cornerstone of an element's identity. It represents the number of protons residing in the nucleus of an atom. For cobalt, Z = 27, signifying the presence of 27 protons.
This number is not merely an identifier; it dictates the number of electrons in a neutral atom. In a neutral cobalt atom, there are precisely 27 electrons orbiting the nucleus. This balance of protons and electrons ensures electrical neutrality.
Electron Orbitals: Defining the Spatial Realm of Electrons
Electrons do not orbit the nucleus in fixed paths like planets around a sun. Instead, they reside in specific regions of space called orbitals. These orbitals are characterized by distinct shapes and energy levels.
The four principal types of atomic orbitals are designated as s, p, d, and f. Each possesses a unique spatial distribution and energy.
Shapes and Energy Levels of Orbitals
-
s orbitals: These are spherical in shape and represent the lowest energy level within a given electron shell. Each shell contains one s orbital.
-
p orbitals: These have a dumbbell shape and are oriented along the x, y, and z axes. Each shell (n ≥ 2) contains three p orbitals, which are degenerate (having the same energy).
-
d orbitals: These possess more complex shapes than s and p orbitals. Each shell (n ≥ 3) contains five d orbitals, also degenerate.
-
f orbitals: These exhibit even more intricate shapes. Each shell (n ≥ 4) contains seven f orbitals, again, all degenerate.
Energy Levels/Shells: Quantized Energy States
Electrons occupy discrete energy levels, also known as electron shells. These shells are designated by the principal quantum number, n, which can be any positive integer (n = 1, 2, 3, 4...).
Higher values of n correspond to higher energy levels and greater average distances from the nucleus. The n = 1 shell is closest to the nucleus and possesses the lowest energy, while the n = 2 shell is further out and has higher energy, and so on.
Sublevels/Subshells: Fine-Tuning Energy
Within each energy level or shell, electrons further differentiate into sublevels, or subshells. These sublevels are denoted by the same letters as the orbitals: s, p, d, and f.
The number of sublevels within a given energy level is equal to the principal quantum number (n). For example, the n = 1 shell has one sublevel (1s), the n = 2 shell has two sublevels (2s and 2p), and the n = 3 shell has three sublevels (3s, 3p, and 3d).
Each sublevel can accommodate a specific number of electrons. An s sublevel can hold a maximum of 2 electrons, a p sublevel can hold 6, a d sublevel can hold 10, and an f sublevel can hold 14.
The Aufbau Principle: Building Electron Configurations
The Aufbau principle (from the German word for "building up") dictates the order in which electrons fill orbitals. This principle states that electrons first occupy the lowest energy orbitals available before filling higher energy ones.
Following the Aufbau principle leads to a predictable sequence of orbital filling. Understanding and applying this principle is paramount.
Hund's Rule: Maximizing Spin Multiplicity
Hund's rule governs the filling of degenerate orbitals (orbitals with the same energy) within a subshell. It states that electrons individually occupy each orbital within a subshell before any orbital is doubly occupied.
Furthermore, all electrons in singly occupied orbitals have the same spin (either spin-up or spin-down). This arrangement maximizes the total spin multiplicity, leading to a more stable electron configuration.
Pauli Exclusion Principle: A Quantum Constraint
The Pauli exclusion principle is a fundamental principle of quantum mechanics. It asserts that no two electrons in an atom can have the same set of four quantum numbers.
This principle has a profound consequence: each atomic orbital can accommodate a maximum of two electrons, and these electrons must have opposite spins. This restriction governs the occupancy of orbitals.
Noble Gas Configuration: A Shorthand Notation
Writing out the full electron configuration can be cumbersome, especially for elements with many electrons. The noble gas configuration offers a convenient shorthand.
It involves representing the electron configuration of the core electrons (those belonging to the preceding noble gas) by the noble gas symbol in brackets, followed by the valence electrons. For example, the noble gas configuration of cobalt will involve using Argon (Ar).
By mastering these fundamental concepts, we equip ourselves with the tools necessary to decipher the electron configuration of cobalt and, consequently, to understand its chemical properties and behavior.
Decoding Cobalt's Electron Configuration: A Step-by-Step Guide
To comprehend the electron configuration of cobalt, a firm grasp of the underlying principles is essential. These fundamental concepts provide the framework for understanding how electrons are arranged within an atom, dictating its chemical behavior and reactivity. Let us delve into the precise, step-by-step method for elucidating Cobalt's electron configuration.
Applying the Aufbau Principle to Cobalt
The Aufbau principle dictates that electrons first occupy the lowest energy levels available. For cobalt (Co), with an atomic number of 27, this means we sequentially fill the orbitals.
Following the order of increasing energy, we arrive at the configuration: 1s² 2s² 2p⁶ 3s² 3p⁶ 4s² 3d⁷.
This sequence represents the ground state electron configuration of cobalt. Each superscript indicates the number of electrons occupying that particular orbital.
It highlights the completion of inner shells before filling the 3d orbitals, which are slightly higher in energy than the 4s orbital.
Hund's Rule and 3d Orbital Filling
Hund's rule becomes crucial when dealing with degenerate orbitals, such as the five 3d orbitals. This rule states that electrons individually occupy each orbital within a subshell before any orbital is doubly occupied. This maximizes spin multiplicity and contributes to greater stability.
In the case of cobalt’s 3d⁷ configuration, five electrons will each occupy a 3d orbital with parallel spins, while the remaining two electrons will pair up in two of the 3d orbitals. This arrangement minimizes electron-electron repulsion.
The final 3d orbital occupancy results in a configuration that is energetically favorable. This influences the magnetic properties of cobalt.
Valence and Core Electrons
Cobalt's electrons are categorized into valence and core electrons. Valence electrons are those in the outermost shell. For cobalt, these are the 4s² electrons.
Valence electrons are primarily involved in chemical bonding and dictate the element's reactivity. Core electrons are all the other electrons.
They reside in the inner shells. Core electrons shield the valence electrons from the full nuclear charge.
This shielding effect impacts the effective nuclear charge experienced by the valence electrons. Effective nuclear charge influences ionization energy and atomic size.
Orbital Diagrams/Box Notation
Orbital diagrams, also known as box notation, provide a visual representation of an atom’s electron configuration. Each box represents an orbital.
Arrows represent electrons, with their direction indicating spin (+1/2 or -1/2). For cobalt, the diagram would show the filling of each orbital.
The 1s, 2s, 2p, 3s, and 3p orbitals are completely filled. The 4s orbital contains two electrons with opposite spins.
The 3d orbitals are represented by five boxes, showing single occupancy for five of them and paired occupancy for the remaining two.
This visual tool reinforces the concepts of Hund's rule and the Pauli exclusion principle. It also enhances understanding of electron distribution within the atom.
Beyond the Basics: Advanced Concepts and Implications for Cobalt
Decoding Cobalt's electron configuration necessitates a firm grasp of the underlying principles. These fundamental concepts provide the framework for understanding how electrons are arranged within an atom, dictating its chemical behavior and reactivity. Let us delve into the advanced implications of Cobalt's configuration.
Cobalt Ions: Electronic Structure and Stability
Cobalt, like many transition metals, exhibits variable valency, forming ions with different charges. The most common ions are Co²⁺ and Co³⁺, which play crucial roles in various chemical compounds and biological systems.
Understanding the electron configurations of these ions is essential for predicting their stability and reactivity. The formation of ions involves the removal of electrons from the outermost shell, typically the 4s and 3d orbitals.
Formation of Co²⁺ Ion
The Co²⁺ ion is formed by removing two electrons from the neutral cobalt atom. The electron configuration of neutral cobalt ([Ar] 4s² 3d⁷) changes to [Ar] 3d⁷ upon ionization. This configuration results in a relatively stable ion due to the partially filled 3d subshell.
Formation of Co³⁺ Ion
The Co³⁺ ion is formed by removing three electrons from the neutral cobalt atom. The electron configuration of the Co³⁺ ion is [Ar] 3d⁶. This configuration is interesting because the 3d⁶ configuration can exhibit both high-spin and low-spin states, depending on the ligand field strength in coordination complexes. The high-spin state has all electrons unpaired, while the low-spin state has electrons paired up.
Magnetic Properties: Paramagnetism of Cobalt
The magnetic properties of cobalt are intimately linked to its electron configuration, specifically the presence of unpaired electrons. Materials with unpaired electrons are paramagnetic, meaning they are attracted to an external magnetic field. Cobalt, in its elemental form and in many of its compounds, exhibits paramagnetism.
The unpaired electrons in the 3d orbitals of cobalt atoms and ions create a net magnetic moment. When an external magnetic field is applied, these magnetic moments align with the field, resulting in a measurable attraction.
The strength of paramagnetism depends on the number of unpaired electrons. For example, both Co²⁺ and Co³⁺ ions, with their respective d⁷ and d⁶ configurations, possess unpaired electrons and are paramagnetic.
Atomic Mass and Isotopes of Cobalt
The atomic mass of an element is the weighted average of the masses of its naturally occurring isotopes. Isotopes are atoms of the same element that have different numbers of neutrons.
While all cobalt atoms have 27 protons, the number of neutrons can vary.
Isotopes of Cobalt
Cobalt has several isotopes, but only one is stable: ⁵⁹Co. ⁵⁹Co makes up nearly 100% of naturally occurring cobalt, giving cobalt a characteristic atomic weight of 58.933 u. Other isotopes, such as ⁶⁰Co, are radioactive and are used in various applications, including medical treatments and industrial radiography.
Relationship Between Atomic Mass and Isotopes
The atomic mass of cobalt is primarily determined by the mass of ⁵⁹Co. The other isotopes, being unstable and present in negligible amounts, do not significantly affect the overall atomic mass. The precise atomic mass reflects the combined mass of protons and neutrons in the nucleus of ⁵⁹Co.
Tools and Resources: Mastering Electron Configuration Analysis
Decoding Cobalt's electron configuration necessitates a firm grasp of the underlying principles. These fundamental concepts provide the framework for understanding how electrons are arranged within an atom, dictating its chemical behavior and reactivity. Let us delve into the advanced tools and resources that will facilitate a deeper comprehension of electron configuration analysis, focusing primarily on the periodic table and electron configuration charts.
The Periodic Table as a Configuration Key
The periodic table is more than just a list of elements; it is a meticulously organized chart that reflects the underlying electronic structure of atoms. Its structure inherently mirrors the filling of electron orbitals. By understanding its organization, one can readily deduce the electron configurations of elements, including Cobalt.
Group and Period Relationships
The periodic table’s layout is deliberate. Elements within the same group (vertical column) exhibit similar chemical properties. This similarity arises because they possess the same number of valence electrons, those in the outermost shell that primarily participate in chemical bonding.
The period (horizontal row) signifies the principal energy level, n, being filled. For example, elements in the fourth period (like Cobalt) have their outermost electrons in the n=4 energy level, involving 4s and 3d orbitals.
Decoding Blocks and Subshells
The periodic table is further divided into blocks corresponding to the filling of specific subshells. The s-block (Groups 1 and 2) represents the filling of the s orbitals. The p-block (Groups 13-18) corresponds to the filling of p orbitals.
The d-block (Transition Metals) accounts for the filling of d orbitals, and the f-block (Lanthanides and Actinides) involves the filling of f orbitals. Cobalt, being a transition metal, resides in the d-block, meaning its valence electrons occupy the d orbitals.
Understanding these block associations significantly simplifies the process of determining the electron configuration. This is because the element's position immediately indicates which subshells are being populated.
Visualizing Electron Configuration: Charts and Diagrams
While the periodic table provides a valuable guide, electron configuration charts and diagrams offer a more direct visual representation of the order in which orbitals are filled. These tools are particularly useful for grasping the Aufbau principle, which dictates the filling of orbitals from lowest to highest energy.
The Aufbau Principle and Diagonal Rule
Electron configuration charts typically illustrate the Aufbau principle through a diagonal rule. This rule visually maps the filling order of orbitals, accounting for the overlap in energy levels between different principal energy levels. For example, the 4s orbital fills before the 3d orbital, despite 3d belonging to a lower principal energy level.
Orbital Box Diagrams
In addition to charts showing filling order, orbital box diagrams provide a detailed depiction of electron occupancy within each orbital. Each box represents an orbital, and arrows represent electrons, with their direction indicating spin. Hund's rule, which states that electrons individually occupy each orbital within a subshell before doubling up in any one orbital, is readily visualized using these diagrams.
For Cobalt, an orbital box diagram would clearly show the distribution of the seven 3d electrons, illustrating that two of the five 3d orbitals are fully occupied, while the remaining three contain a single electron each, all with the same spin initially to maximize total spin.
Video: Cobalt Electron Configuration: Guide for Students
Frequently Asked Questions
Why is the electron configuration of cobalt sometimes written with the 4s orbital filled before the 3d orbitals?
Although electrons fill the 4s orbital before the 3d orbital, the 3d orbitals are slightly lower in energy once they are occupied. Therefore, the standard way to represent the cobalt electron configuration is [Ar] 3d⁷ 4s², reflecting the actual energy levels of the electrons.
What is the abbreviated or noble gas configuration for cobalt?
The abbreviated or noble gas electron configuration for cobalt is [Ar] 3d⁷ 4s². This notation replaces the long, full electron configuration with the symbol of the preceding noble gas (Argon, [Ar]) and then lists only the electrons in the outermost shells beyond that.
How does the electron configuration of cobalt relate to its chemical properties?
Cobalt's electron configuration, specifically the presence of seven electrons in its 3d orbitals and two in its 4s orbital, significantly influences its chemical properties. These electrons are involved in forming chemical bonds and determine its oxidation states and ability to form complexes.
Is the electron configuration of cobalt the same for the cobalt(II) ion (Co²⁺)?
No. When cobalt forms the Co²⁺ ion, it loses two electrons. These are removed from the outermost shell, resulting in the electron configuration of [Ar] 3d⁷. Therefore, the cobalt electron configuration for the ion differs from the neutral atom.
So, there you have it! Hopefully, this guide has demystified the cobalt electron configuration for you. Keep practicing, and soon you'll be a pro at predicting the electron configurations of other elements too. Good luck with your studies!