ClO Lewis Structure: Easy Steps & The Shocking Truth!
Understanding the clo - lewis structure can be simplified by recognizing its connection to fundamental chemistry concepts. The octet rule, a cornerstone of chemical bonding, directly influences how we arrange electrons in the ClO molecule. VSEPR theory, another essential tool, helps us predict the molecular geometry once the Lewis structure is established. Researchers at the National Institute of Standards and Technology (NIST) frequently utilize spectroscopic data to validate such structural predictions. Finally, understanding the electronegativity differences, a principle championed by Linus Pauling, is critical in determining the polarity of the Cl-O bond within the clo - lewis structure.
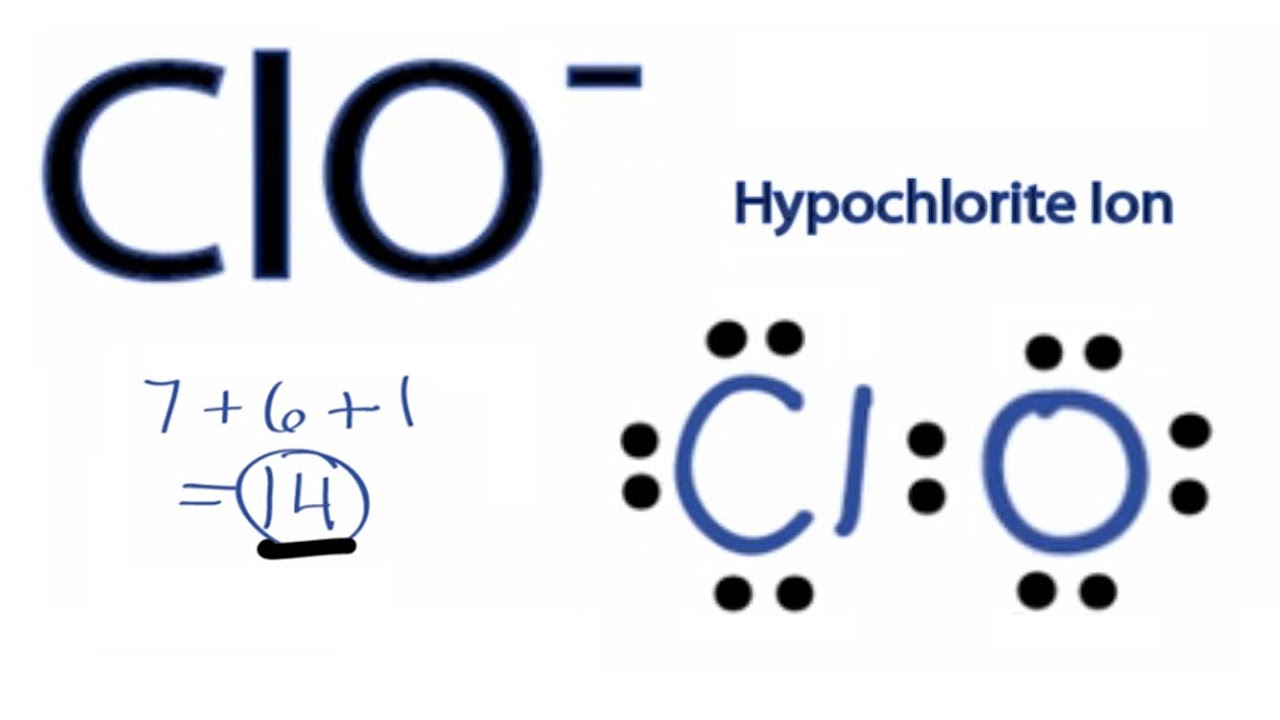
Image taken from the YouTube channel Wayne Breslyn (Dr. B.) , from the video titled ClO- Lewis Structure - How to Draw the Lewis Structure for ClO- .
Unveiling the Enigmatic ClO Lewis Structure
The Lewis structure of chlorine monoxide (ClO) might seem like a straightforward exercise in applying chemical bonding principles. However, this seemingly simple molecule holds a secret, a "shocking truth" that unveils the complexities and nuances often hidden beneath the surface of basic chemical representations. Understanding the ClO Lewis structure isn't just about drawing lines and dots; it's about grasping the very essence of chemical bonding and reactivity.
The Significance of Lewis Structures
Lewis structures are fundamental tools in chemistry.
They allow us to visualize the arrangement of atoms within a molecule and, more importantly, to understand how electrons are distributed.
This distribution of electrons dictates a molecule's properties, its reactivity, and its interactions with other molecules.
The ClO Lewis structure is a particularly insightful case study, demonstrating both the power and the limitations of this fundamental concept.
ClO: More Than Meets the Eye
Chlorine monoxide, at first glance, appears to be a simple diatomic molecule composed of one chlorine atom and one oxygen atom.
However, ClO is a free radical, a highly reactive species with an unpaired electron. This single fact makes its Lewis structure particularly interesting and somewhat challenging to represent accurately.
The typical rules we learn for drawing Lewis structures, such as satisfying the octet rule, don't always neatly apply to molecules like ClO.
This "shocking truth"—that ClO is a free radical—has profound implications for its chemistry.
It is exceptionally reactive and plays a critical role in atmospheric processes, particularly ozone depletion.
What We Will Cover
In this exploration of the ClO Lewis structure, we will embark on a journey to fully understand this captivating molecule.
First, we will cover the fundamentals of Lewis structures and valence electrons, ensuring a solid foundation for our analysis.
We will then proceed with a step-by-step guide on how to construct the ClO Lewis structure, highlighting the challenges and considerations that arise due to its free-radical nature.
Finally, we will reveal the "shocking truth" about ClO's identity and discuss its implications, exploring the role of resonance and the limitations of the octet rule in accurately representing this fascinating molecule.
Unveiling the role of chlorine monoxide as a critical player in atmospheric chemistry and chemical bonding compels us to scrutinize its structure. Before diving into the complexities of the ClO Lewis structure itself, it's crucial to establish a solid foundation in the underlying principles.
Fundamentals: Setting the Stage for ClO's Structure
Lewis structures are essential tools in a chemist's arsenal.
They provide a visual representation of molecules, revealing how atoms are connected and how electrons are distributed.
But what exactly are Lewis structures, and why are they so important?
Defining Lewis Structures and Their Purpose
A Lewis structure, also known as an electron dot diagram, is a diagram that shows the bonding between atoms of a molecule, as well as any lone pairs of electrons that may exist.
These diagrams use dots to represent valence electrons.
Valence electrons are the outermost electrons of an atom that participate in chemical bonding.
Lewis structures help us to:
- Visualize molecular geometry: Getting a sense of how atoms are arranged in space.
- Predict molecular properties: Including reactivity and polarity.
- Understand chemical bonding: Differentiating between single, double, and triple bonds.
Introducing Chlorine (Cl) and Oxygen (O)
Chlorine monoxide (ClO), as its name suggests, is composed of two key elements: chlorine (Cl) and oxygen (O).
Understanding the individual properties of these elements is crucial to understanding the molecule they form together.
Chlorine is a halogen, a highly reactive nonmetal known for its ability to readily form chemical bonds.
Oxygen is another highly reactive nonmetal, essential for life and a key player in combustion and oxidation reactions.
Their individual electron configurations dictate how they will interact to form ClO.
Valence Electrons: The Basis of Chemical Bonding
The concept of valence electrons is central to understanding how atoms bond.
Valence electrons are the electrons in the outermost shell of an atom and are the ones involved in forming chemical bonds.
The number of valence electrons an atom possesses dictates its bonding behavior.
Atoms "want" to achieve a stable electron configuration, often resembling that of a noble gas, which typically involves having eight valence electrons (octet rule).
Atoms achieve this stability by sharing, donating, or accepting valence electrons through chemical bonding.
Determining the Number of Valence Electrons for Cl and O
To draw an accurate Lewis structure, we must first know the number of valence electrons for each atom in the molecule.
Here's how to determine that:
-
Chlorine (Cl): Chlorine is in Group 17 (also known as VIIA) of the periodic table.
Atoms in Group 17 have seven valence electrons.
-
Oxygen (O): Oxygen resides in Group 16 (VIA) of the periodic table.
Therefore, oxygen has six valence electrons.
These values are essential for constructing the ClO Lewis structure in the subsequent section. Accurately determining and representing valence electrons is the first critical step to unveil the "shocking truth" hidden in ClO.
Fundamentals established, elements introduced – now the real work begins. Understanding the individual components is essential, but the magic truly happens when they combine. We are now equipped to delve into the practical application of Lewis structure principles to the ClO molecule itself, guiding you through each step of the construction process.
Step-by-Step Guide: Constructing the ClO Lewis Structure
Drawing a Lewis structure might seem daunting at first, but breaking it down into manageable steps makes the process straightforward. Follow these steps, and you'll be able to confidently represent the electronic structure of chlorine monoxide.
Step 1: Calculate Total Valence Electrons in ClO
The first, and arguably most important, step is to determine the total number of valence electrons present in the molecule. This dictates how many electrons you have to work with when forming bonds and lone pairs.
Remember, valence electrons are the electrons in the outermost shell of an atom. These are the electrons involved in chemical bonding.
To find the total, add the number of valence electrons for each atom in the molecule.
- Chlorine (Cl) is in group 17 (or 7A), so it has 7 valence electrons.
- Oxygen (O) is in group 16 (or 6A), so it has 6 valence electrons.
Therefore, the total number of valence electrons in ClO is 7 + 6 = 13 valence electrons.
This odd number immediately hints at something unusual about the ClO molecule.
Step 2: Draw the Skeletal Structure
Next, draw the basic framework of the molecule. This involves connecting the atoms with single bonds. For ClO, this is simple: connect the chlorine atom to the oxygen atom with a single line, representing a single covalent bond.
Cl – O
This single bond represents two shared electrons, one from each atom.
While the structure itself is straightforward here, remember that in more complex molecules, the least electronegative atom usually occupies the central position.
Step 3: Distribute Remaining Valence Electrons as Lone Pairs
Now, distribute the remaining valence electrons as lone pairs around the atoms. Lone pairs are pairs of electrons that are not involved in bonding and are represented as dots around the atom's symbol.
The goal here is to satisfy the octet rule, which states that atoms "want" to be surrounded by eight valence electrons. Hydrogen is an exception and only needs two.
Start by distributing electrons around the more electronegative atom (oxygen in this case) until it has an octet.
After giving oxygen six electrons as lone pairs (three pairs), that atom has eight electrons total (six lone pair electrons, and 2 from the bond with chlorine).
This leaves us with 13 (total) - 6 (on O) - 2 (in the bond) = 5 electrons to distribute.
Place the remaining five electrons around the chlorine atom.
This gives chlorine a total of seven electrons (five lone pair electrons, and two from the bond).
At this point the structure should look something like this:
.. .. : Cl – O : . ..
Step 4: Calculate Formal Charge on Each Atom
Formal charge helps to determine the most stable Lewis structure when multiple possibilities exist. It's the hypothetical charge an atom would have if all bonding electrons were shared equally between the atoms.
The formula for calculating formal charge is:
Formal Charge = (Valence Electrons) – (Non-bonding Electrons + 1/2 Bonding Electrons)
- For Chlorine: Formal Charge = 7 – (5 + 1/2
**2) = 7 – 6 = +1
- For Oxygen: Formal Charge = 6 – (6 + 1/2** 2) = 6 – 7 = -1
Step 5: Evaluate Octet Rule Satisfaction
Now, assess how well the octet rule is satisfied. In our initial structure, oxygen has a complete octet, but chlorine only has seven electrons. This is where the "shocking truth" about ClO comes into play, which we'll discuss in the next section.
The Lewis structure we've drawn is a valid representation, but it highlights the inherent instability of the ClO molecule due to the unpaired electron on chlorine.
Fundamentals established, elements introduced – now the real work begins. Understanding the individual components is essential, but the magic truly happens when they combine. We are now equipped to delve into the practical application of Lewis structure principles to the ClO molecule itself, guiding you through each step of the construction process.
The Shocking Truth: ClO's Identity as a Free Radical
After meticulously working through the steps of constructing the ClO Lewis structure, you might notice something unusual. It becomes apparent that achieving a perfect octet for both chlorine and oxygen simultaneously is impossible. This impossibility reveals a fundamental truth about chlorine monoxide: it's a free radical.
But what does it mean for ClO to be a free radical, and why is it so significant?
Unveiling the Unpaired Electron
The defining characteristic of a free radical is the presence of one or more unpaired electrons. In the case of ClO, the total number of valence electrons (13) is odd. This odd number dictates that at least one atom within the molecule will inevitably possess an unpaired electron.
This unpaired electron makes ClO highly reactive, setting it apart from molecules with complete electron pairing.
The Frustration of the Octet Rule
The drive to satisfy the octet rule – the tendency of atoms to achieve a stable configuration of eight valence electrons – governs much of chemical bonding. However, ClO presents a challenge to this rule. Due to the odd number of valence electrons, one atom will be left with an incomplete octet, regardless of how the electrons are arranged.
This inherent instability, caused by the unpaired electron, is at the heart of ClO's identity as a free radical.
Implications of Being a Free Radical
The consequences of ClO's free radical nature are profound, especially in terms of its reactivity and environmental impact.
High Reactivity: A Chemical Agitator
Free radicals, by their very nature, are highly reactive. The unpaired electron seeks to pair up, leading ClO to readily participate in chemical reactions. This eagerness to react allows ClO to interact with a wide range of molecules.
Role in Atmospheric Chemistry: The Ozone Depletion Connection
ClO's reactivity plays a crucial role in atmospheric chemistry, particularly concerning ozone depletion. In the stratosphere, ClO acts as a catalyst in the breakdown of ozone (O3) molecules.
Here's a simplified view:
- ClO reacts with O3, forming ClOO and O2.
- ClOO rapidly decomposes, releasing Cl and O2.
- The Cl atom then reacts with another O3 molecule, repeating the cycle.
This catalytic cycle can destroy thousands of ozone molecules before ClO is removed from the stratosphere, contributing significantly to the depletion of the ozone layer, which protects us from harmful UV radiation.
Fundamentals established, elements introduced – now the real work begins. Understanding the individual components is essential, but the magic truly happens when they combine. We are now equipped to delve into the practical application of Lewis structure principles to the ClO molecule itself, guiding you through each step of the construction process.
The Shocking Truth: ClO's Identity as a Free Radical
After meticulously working through the steps of constructing the ClO Lewis structure, you might notice something unusual. It becomes apparent that achieving a perfect octet for both chlorine and oxygen simultaneously is impossible. This impossibility reveals a fundamental truth about chlorine monoxide: it's a free radical.
But what does it mean for ClO to be a free radical, and why is it so significant?
Unveiling the Unpaired Electron
The defining characteristic of a free radical is the presence of one or more unpaired electrons. In the case of ClO, the total number of valence electrons (13) is odd. This odd number dictates that at least one atom within the molecule will inevitably possess an unpaired electron.
This unpaired electron makes ClO highly reactive, setting it apart from molecules with complete electron pairing.
The Frustration of the Octet Rule
The drive to satisfy the octet rule – the tendency of atoms to achieve a stable configuration of eight valence electrons – governs much of chemical bonding. However, ClO presents a challenge to this rule. Due to the odd number of valence electrons, one atom will be left with an incomplete octet.
This leads us to consider that the Lewis structure we derived is merely one representation of the molecule. So how do we deal with this imperfection?
Dealing with Imperfections: Resonance and Limitations
The Lewis structure provides a powerful framework for understanding chemical bonding. However, it is essential to recognize its limitations. In some cases, a single Lewis structure cannot accurately represent the electron distribution in a molecule. ClO exemplifies this scenario, forcing us to consider alternative representations and the nuances of the octet rule.
Resonance Structures: Acknowledging the Ambiguity
For molecules like ClO, where a single Lewis structure falls short, the concept of resonance structures comes into play. Resonance structures are two or more Lewis structures that can represent the same molecule. They differ only in the distribution of electrons, not in the arrangement of atoms.
The actual electronic structure of the molecule is a hybrid, or weighted average, of these resonance structures.
Illustrating Alternative ClO Structures
In the case of ClO, we can draw at least two significant resonance structures. In one, the unpaired electron resides on the chlorine atom, while in the other, it's on the oxygen atom. Both structures contribute to the overall picture of the molecule's electronic distribution.
The true structure of ClO is neither of these individual structures, but rather a blend of the two. This blending is what stabilizes the molecule.
Electron Dot Diagram: Visualizing Valence Electrons
The electron dot diagram, also known as a Lewis dot diagram, is a simplified way to visually represent the valence electrons in an atom or molecule. Instead of drawing lines to represent bonds, dots are used to depict the valence electrons around each atom.
This diagram can be useful for quickly visualizing the number of valence electrons and the presence of unpaired electrons in molecules like ClO.
The Octet Rule: When Rules Are Meant to Be Broken
The octet rule serves as a valuable guideline, but it is not an absolute law. Many molecules, particularly those involving elements in the third row and beyond of the periodic table, can violate the octet rule. In these cases, atoms may have more or fewer than eight electrons in their valence shell.
ClO, being a free radical, inherently defies the octet rule. The odd number of valence electrons makes it impossible for both chlorine and oxygen to achieve a complete octet simultaneously.
Formal Charge: Guiding Structure Selection
While resonance structures help depict electron delocalization, not all resonance structures are created equal. The concept of formal charge helps us determine which resonance structure is the most plausible and contributes most significantly to the overall structure of the molecule.
Formal charge is the hypothetical charge an atom would have if all bonding electrons were shared equally between the atoms.
Formal Charge and Stability
The resonance structure with the smallest formal charges, and with negative formal charges on the more electronegative atoms, is generally considered to be the most stable and the major contributor to the true structure of the molecule. Analyzing the formal charges on chlorine and oxygen in the different resonance structures of ClO provides further insight into the molecule's electronic behavior.
Video: ClO Lewis Structure: Easy Steps & The Shocking Truth!
FAQs: ClO Lewis Structure - Unveiling the Truth
[This section clarifies common questions about drawing the ClO Lewis structure and understanding its properties.]
Why is the ClO Lewis structure sometimes considered "shocking"?
The "shocking" aspect often refers to the fact that ClO (hypochlorite ion) carries a negative charge and also that chlorine sometimes expands its octet, although in the simplest representation of the clo - lewis structure, it doesn't need to. It demonstrates how seemingly simple molecules can have nuanced electronic arrangements.
What are the key steps for drawing the ClO Lewis structure?
First, count valence electrons (6 from O, 7 from Cl, +1 for the negative charge = 14). Then, connect the atoms with a single bond. Distribute remaining electrons as lone pairs, prioritizing the more electronegative oxygen. Ensure formal charges are minimized.
Is there more than one valid Lewis structure for ClO?
While the most common clo - lewis structure shows a single bond between chlorine and oxygen with three lone pairs on each, resonance structures can exist, though they are generally less significant due to charge separation. The key is adhering to valence electron count and minimizing formal charges.
What does the ClO Lewis structure tell us about its reactivity?
The ClO Lewis structure shows that the ion carries a negative charge and has available lone pairs on oxygen and chlorine. This makes it a powerful oxidizing agent used in bleach and disinfectants, as it readily accepts electrons from other substances. The clo - lewis structure visually supports this understanding of its reactivity.