Chromium Electron Configuration: A Detailed Guide
Chromium, an element often studied in the context of inorganic chemistry, exhibits a unique electronic structure influencing its chemical behavior. The National Institute of Standards and Technology (NIST) provides extensive data on atomic properties, including details relevant to chromium electron configuration. Understanding the electron configuration of chromium is crucial for predicting its reactivity and the formation of various compounds, such as those used in catalysis. Hund's rule, a principle in quantum mechanics, helps to explain the distribution of electrons in chromium's orbitals, accounting for its stability and distinct electron arrangement.
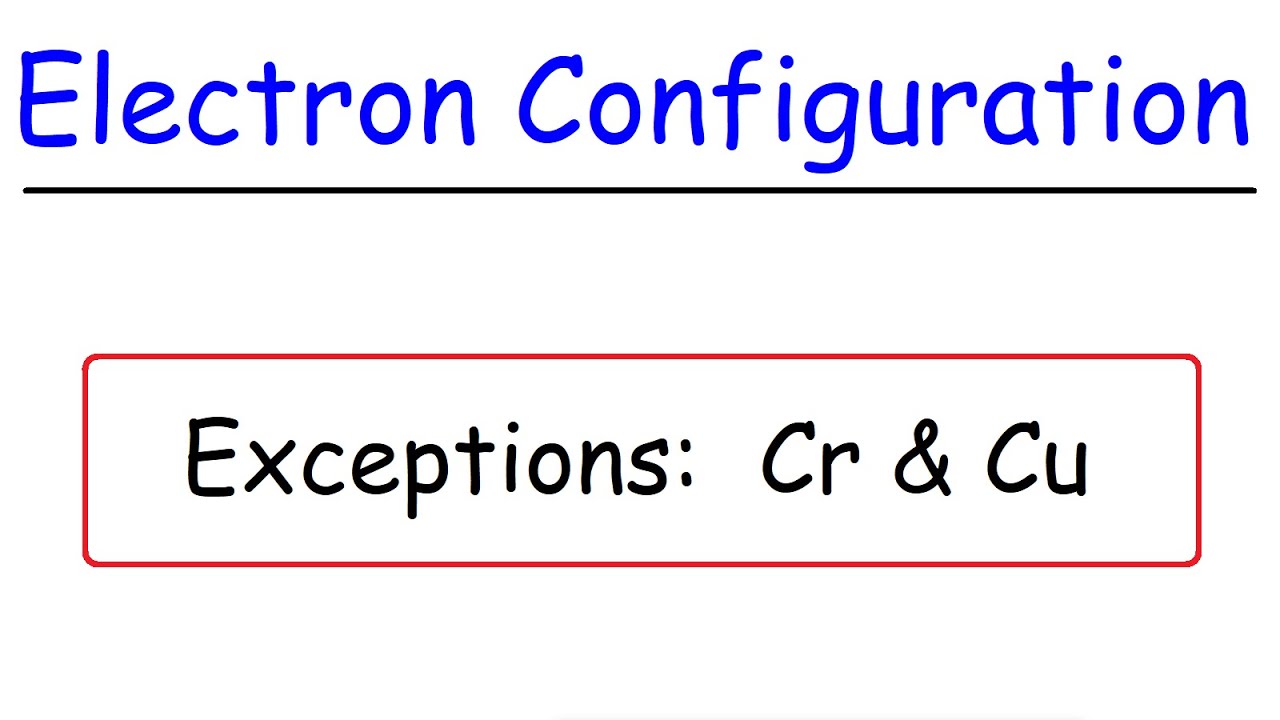
Image taken from the YouTube channel The Organic Chemistry Tutor , from the video titled Electron Configuration Exceptions - Chromium (Cr) & Copper (Cu) .
Unveiling the Enigma: The Electron Configuration of Chromium
The arrangement of electrons within an atom, known as its electron configuration, dictates its chemical behavior. This arrangement dictates how an atom interacts with other atoms, forming the bonds that create the molecules and compounds that constitute our world.
Electron configuration, in essence, is the address of each electron within an atom. It determines its chemical properties and reactivity. Understanding electron configurations is therefore paramount to understanding chemical reactions and material properties.
Chromium: A Transition Metal of Industrial Significance
Chromium (Cr), a hard, lustrous, steel-gray transition metal, holds a prominent position in numerous industrial applications. Its resistance to corrosion makes it an essential component in stainless steel. Chromium is also critical to the creation of durable alloys and pigments.
Beyond its industrial applications, Chromium plays a vital role in biological systems, such as glucose metabolism and insulin signaling. Its versatility underscores the importance of understanding its fundamental properties.
The Anomalous Electron Configuration of Chromium: A Deviation from Expectations
While the principles governing electron configuration generally hold true, there are notable exceptions. Chromium presents one such exception.
Based on the Aufbau principle, we would expect Chromium to have an electron configuration of [Ar] 4s2 3d4. However, experimental evidence reveals a different configuration: [Ar] 4s1 3d5.
This seemingly minor deviation unveils a fascinating aspect of atomic structure. The 4s1 3d5 configuration exhibits an unexpected stability that defies simple application of the Aufbau principle.
The primary purpose of this discussion is to dissect the underlying reasons behind this anomaly. We aim to explain why Chromium adopts this unique electron configuration.
Fundamental Principles: Building Blocks of Electron Configuration
Unveiling the Enigma: The Electron Configuration of Chromium The arrangement of electrons within an atom, known as its electron configuration, dictates its chemical behavior. This arrangement dictates how an atom interacts with other atoms, forming the bonds that create the molecules and compounds that constitute our world.
Electron configuration, governed by fundamental principles, provides the framework for understanding the chemical properties of elements. Before we can appreciate the anomaly of Chromium’s electron configuration, we must first establish a firm grasp on these foundational concepts.
Defining the Atomic Number (Z)
The atomic number, represented by the symbol Z, is the cornerstone of an element's identity. It is defined as the number of protons found within the nucleus of an atom. This number is unique for each element, distinguishing it from all others.
For example, Chromium has an atomic number of 24, meaning every Chromium atom contains precisely 24 protons in its nucleus. The atomic number dictates the element's position on the periodic table and serves as the basis for understanding its electronic structure.
The Guiding Hand: The Aufbau Principle
The Aufbau principle, derived from the German word "Aufbauen" meaning "to build up," dictates the order in which electrons fill atomic orbitals. Electrons initially occupy the lowest energy levels available before populating higher energy levels.
This principle provides a systematic approach to predicting electron configurations. It follows a specific sequence, often visualized using the "diagonal rule," which outlines the filling order of orbitals (1s, 2s, 2p, 3s, 3p, 4s, 3d, etc.).
However, the Aufbau principle is not without its exceptions, as we will see with Chromium. These exceptions underscore the complexities of electron interactions and the stability associated with certain electronic arrangements.
Maximizing Spin Multiplicity: Hund's Rule
Hund's rule provides specific guidance on filling degenerate orbitals (orbitals with the same energy level) within a subshell. It states that electrons will individually occupy each orbital within a subshell before doubling up in any one orbital.
Furthermore, these unpaired electrons will have the same spin (either spin-up or spin-down), maximizing the total spin multiplicity. This maximizes the stability of the electron configuration. This rule is a direct consequence of quantum mechanical exchange energy.
For instance, when filling the three p orbitals (px, py, pz), electrons will first occupy each orbital singly with parallel spins before any orbital receives a second electron.
The Exclusionary Principle: Pauli's Decree
The Pauli Exclusion Principle is a fundamental tenet of quantum mechanics. It asserts that no two electrons within an atom can possess the identical set of four quantum numbers (n, l, ml, ms).
This principle directly implies that each atomic orbital can hold a maximum of only two electrons, and these electrons must have opposite spins (+1/2 and -1/2). This restriction is crucial for determining the maximum number of electrons that can occupy a given energy level or subshell.
Defining Energy Levels (n)
Energy levels, denoted by the principal quantum number "n," represent the quantized energy states that electrons can occupy within an atom. Higher values of "n" indicate higher energy levels.
These energy levels correspond to the electron shells surrounding the nucleus. As "n" increases (n = 1, 2, 3, etc.), electrons are located further from the nucleus and possess greater potential energy.
Orbitals: Probability Landscapes
Orbitals are mathematical functions that describe the probability of finding an electron in a specific region of space around the nucleus. They are not physical pathways but rather represent the electron's probable distribution.
Orbitals are categorized by their shapes, which are designated by the letters s, p, d, and f. Each type of orbital has a distinct spatial orientation and energy level.
- s orbitals: Spherical in shape.
- p orbitals: Dumbbell-shaped and oriented along the x, y, and z axes.
- d orbitals: More complex shapes with various spatial orientations.
- f orbitals: Even more intricate shapes.
Shells and Subshells: Organizing Electron Distribution
Subshells are groups of orbitals within a given energy level (n) that share the same shape (s, p, d, or f). For example, the n = 2 energy level contains two subshells: 2s and 2p.
The arrangement of electrons into these subshells determines the electron configuration of an atom. It is the filling pattern of these subshells that dictates many of the element's chemical and physical properties. The interplay of these principles provides the foundation for understanding and predicting the electron configurations of elements, and the exceptions to these rules, like Chromium, highlight the intricate nature of electron behavior.
The Chromium Anomaly: Expected vs. Observed Configurations
The arrangement of electrons within an atom, known as its electron configuration, dictates its chemical behavior. This arrangement dictates how an atom interacts with other atoms, forming the bonds that create the molecules that make up the substance of the universe.
Understanding the rules and principles guiding electron configuration is essential for making accurate predictions about the properties of various elements. However, in the realm of transition metals, these predictions do not always align with experimental observations.
Chromium (Cr), with its atomic number of 24, presents a notable deviation from the expected electron configuration, an anomaly that has intrigued chemists and physicists alike.
The Predicted Configuration: Following the Aufbau Principle
Based on the Aufbau principle, which dictates that electrons first occupy the lowest energy levels available, one would anticipate the electron configuration of Chromium to be:
1s2 2s2 2p6 3s2 3p6 4s2 3d4.
This arrangement adheres to the filling order dictated by the relative energies of the orbitals, sequentially filling the s and p orbitals before proceeding to the d orbitals.
Thus, the 4s orbital, being lower in energy than the 3d orbitals in this simplistic model, is expected to fill completely before any electrons occupy the 3d orbitals.
The Observed Configuration: An Unexpected Arrangement
Experimental evidence, derived from spectroscopic studies and other analytical techniques, reveals a different reality. The true electron configuration of Chromium is:
1s2 2s2 2p6 3s2 3p6 4s1 3d5.
This observed configuration indicates that one electron from the 4s orbital has shifted to the 3d orbital, resulting in a half-filled 4s orbital and a half-filled 3d subshell.
The Core Anomaly: 4s1 3d5 vs. 4s2 3d4
The discrepancy between the expected (4s2 3d4) and observed (4s1 3d5) configurations constitutes the Chromium anomaly.
This deviation from the predicted electron configuration challenges the direct application of the Aufbau principle and necessitates a deeper examination of the factors governing electronic stability within atoms.
This rearrangement of electrons is not arbitrary; it reflects a system's inherent drive to achieve a state of lower energy and greater stability.
Understanding why Chromium adopts this seemingly unconventional configuration is key to unraveling the nuances of electron behavior and predicting the properties of other transition metals.
Unraveling the Mystery: Factors Contributing to the Anomaly
[The Chromium Anomaly: Expected vs. Observed Configurations The arrangement of electrons within an atom, known as its electron configuration, dictates its chemical behavior. This arrangement dictates how an atom interacts with other atoms, forming the bonds that create the molecules that make up the substance of the universe. Understanding the rules...]
Understanding the anomaly of Chromium's electron configuration requires a deeper dive into the subtle interplay of energetic factors that govern electron distribution. It's not merely about blindly following the Aufbau principle. Several key factors contribute to the observed 4s1 3d5 configuration.
The driving force behind this deviation stems from the enhanced stability associated with half-filled and fully-filled d-orbitals.
The Stability of Half-Filled d-Orbitals
An electronic configuration with a half-filled d-subshell is more stable than one that is partially filled but not half-filled. In Chromium's case, promoting an electron from the 4s orbital to the 3d orbital results in a half-filled 3d subshell (3d5), which is associated with an increase in overall stability.
This stability arises from a complex interplay of factors. These include reduced electron-electron repulsion and enhanced exchange energy, which we will explore further.
Exchange Energy: Minimizing Electron Repulsion
Exchange energy is a quantum mechanical effect that arises from the indistinguishability of electrons. It manifests as a stabilization energy when electrons with parallel spins occupy degenerate orbitals.
In the 4s1 3d5 configuration, all six valence electrons (one in 4s and five in 3d) have parallel spins. This maximizes the exchange energy. The energy gained from this exchange offsets the energy cost of promoting an electron from the 4s to the 3d orbital.
Spherically Symmetrical Charge Distribution and its Impact
The 4s1 3d5 configuration also leads to a more spherically symmetrical distribution of electron density around the nucleus. This enhanced symmetry reduces electron-electron repulsion.
A more even distribution of charge minimizes the overall potential energy of the system. Leading to a more stable arrangement.
The Drive Towards a Lower Energy State
Ultimately, an atom will adopt the electron configuration that minimizes its total energy. While the Aufbau principle provides a useful guideline, it is an approximation.
The actual electron configuration is determined by the complex balance of all energetic contributions. In Chromium, the stability gained from having a half-filled d-orbital, the maximization of exchange energy, and the reduction of electron-electron repulsion collectively outweigh the energy cost of promoting an electron from the 4s orbital.
This results in the observed 4s1 3d5 configuration, which represents a lower energy state for the Chromium atom. The atom seeks to be at the minimum possible energy and the deviation is a testament to this fundamental principle.
Chromium in Context: A Comparative Look at Electron Configurations
The unique electron configuration of Chromium, with its single 4s electron and half-filled 3d subshell, is not an isolated phenomenon within the periodic table. To fully appreciate the factors driving this anomaly, it's crucial to examine how it compares to other transition metals, particularly those in its group and period. This comparative analysis reveals underlying trends and highlights the delicate balance of energetic considerations that dictate electron arrangements.
General Trends Among Transition Metals
Transition metals, characterized by their partially filled d-orbitals, often exhibit variable oxidation states and complex chemical behavior. Their electron configurations generally follow the Aufbau principle, but deviations are not uncommon, especially in the later periods. These deviations arise from the relatively small energy difference between the ns and (n-1)d orbitals, making the overall energy balance of electron pairing, exchange energy, and shielding effects crucial.
The filling of d orbitals across a period leads to increased nuclear charge and a stronger attraction for electrons. However, the d electrons also shield the outer s electrons, impacting their energy levels. This interplay results in subtle energetic shifts that can favor unconventional electron configurations.
Chromium vs. Manganese: A Tale of Two Neighbors
Directly adjacent to Chromium in the periodic table lies Manganese (Mn), with an atomic number of 25. Its expected electron configuration is [Ar] 4s2 3d5. Unlike Chromium, Manganese adheres to the expected configuration, with a completely half-filled 3d subshell and a filled 4s subshell.
The key difference lies in the energetic gain achieved by Chromium through the promotion of a 4s electron to the 3d subshell. This results in a symmetrical 3d5 configuration. While Manganese also has the stable 3d5 arrangement, it already possesses two electrons in the 4s orbital, making the energetic benefit of further electron promotion insufficient to overcome the pairing energy in the 3d subshell.
The Copper Anomaly: A Parallel Case
Chromium is not alone in its deviation from the expected electron configuration. Copper (Cu), further along in the transition metal series, exhibits a similar anomaly. Instead of the expected [Ar] 4s2 3d9, Copper adopts the configuration [Ar] 4s1 3d10, resulting in a completely filled d-orbital.
This parallel illustrates a broader principle: the stability associated with completely filled or half-filled d orbitals can outweigh the energetic cost of promoting an s electron. The d10 configuration in Copper represents a particularly stable state, overcoming the slight energetic penalty of having only one electron in the 4s orbital. This configuration optimizes electron pairing and minimizes electron-electron repulsion.
Group 6 Elements: A Family Affair
Chromium belongs to Group 6 of the periodic table, which also includes Molybdenum (Mo), Tungsten (W), and Seaborgium (Sg). Molybdenum follows a similar pattern to Chromium, adopting an electron configuration of [Kr] 5s1 4d5.
Tungsten, on the other hand, adheres to the expected configuration of [Xe] 6s2 4f14 5d4. The increased number of electrons and the presence of the 4f subshell in Tungsten introduces additional factors that influence the energetic balance, preventing a similar electron promotion. Seaborgium, being a synthetic element, has not been fully characterized, but theoretical calculations suggest it may follow Tungsten's configuration.
The trends within Group 6 highlight the fact that while the principle of half-filled and filled subshells promotes stability, its manifestation varies across the periodic table, depending on the specific energetic landscape of each element. These variations underscore the complexity of electron configurations in transition metals and the importance of considering multiple factors to fully understand observed configurations.
The Consequences: How the Anomaly Shapes Chromium's Behavior
The unique electron configuration of Chromium, with its single 4s electron and half-filled 3d subshell, is not merely an academic curiosity. Its direct influence manifests in a distinctive chemical behavior, molding its interactions with other elements and dictating the stability and properties of its diverse compounds. Understanding this configuration is, therefore, paramount to comprehending Chromium's role in chemical reactions and material science.
Impact on Chemical Properties and Bonding
The singular 4s1 3d5 configuration dictates Chromium's versatile bonding behavior.
Its capacity to readily lose electrons allows it to exhibit a range of oxidation states, most notably +2, +3, and +6, each imparting distinct characteristics to its compounds. The availability of six valence electrons, arising from the single 4s and five 3d electrons, facilitates diverse bonding arrangements. This is critical in applications from catalysis to structural materials.
The stability conferred by the half-filled d-orbital arrangement also influences the geometry and reactivity of Chromium complexes. The electron configuration facilitates efficient ligand field stabilization in certain coordination geometries.
This can make specific Chromium complexes particularly stable and resistant to unwanted reactions.
Formation of Common Chromium Ions (Cr2+, Cr3+, Cr6+)
Chromium's chemical versatility is prominently displayed in the formation of several important ions, each displaying distinctive properties and functions.
Chromium(II) Ion (Cr2+)
The Cr2+ ion arises from the loss of the two 4s1 electrons.
In aqueous solution, Cr2+ is a powerful reducing agent, readily oxidized to Cr3+. The resulting electron configuration is [Ar]3d4, which is highly susceptible to oxidation due to the tendency to achieve the more stable half-filled d-orbital configuration of Cr3+.
Chromium(III) Ion (Cr3+)
Cr3+ is arguably the most stable and common ion of Chromium. It originates from the loss of the 4s1 and two 3d electrons, or oxidation of Cr2+.
The electron configuration is [Ar]3d3. This gives it a half-filled t2g orbital set in octahedral complexes, and contributes to substantial stability in coordination compounds. It is also the basis for the vibrant green color often associated with Chromium compounds.
Chromium(VI) Species (CrO42-, Cr2O72-)
In highly oxidizing conditions, Chromium can lose all six valence electrons to form Chromium(VI) species, predominantly as chromate (CrO42-) and dichromate (Cr2O72-) ions.
These ions are powerful oxidizing agents. The high oxidation state and resulting electron configuration (isoelectronic with Argon) destabilizes these species, making them readily reduced. They are also implicated in toxicity concerns, particularly with Cr(VI) compounds.
The equilibrium between chromate and dichromate is pH-dependent. Chromate predominates under alkaline conditions, while dichromate is favored in acidic environments.
Properties of Important Chromium Compounds
Chromium forms numerous compounds. Their properties reflect the element’s unique electron configuration and ability to exist in multiple oxidation states.
Chromium(III) Oxide (Cr2O3)
Chromium(III) oxide is a stable, green pigment widely used in paints, ceramics, and metallurgy. It is an amphoteric oxide, meaning it can react with both acids and bases, albeit with difficulty.
Its inertness and high melting point make it valuable as a protective coating on metals, contributing to corrosion resistance.
Potassium Dichromate (K2Cr2O7)
Potassium dichromate is a strong oxidizing agent. In laboratory settings, it is used in titrations and various chemical reactions.
Industrially, it serves in leather tanning, wood preservation, and the production of other Chromium compounds. However, due to the toxicity of Cr(VI) compounds, its use is increasingly restricted.
In summary, Chromium's unique electron configuration underpins its versatile chemistry. The stability of its half-filled d-orbital influences its ionic states, the properties of its oxides, and the behavior of its compounds, impacting a wide array of applications. A thorough appreciation of this electronic structure is crucial for the responsible use and development of Chromium-based materials.
Experimental Evidence: Unveiling Chromium's Electronic Structure
The seemingly abstract concept of electron configuration gains tangible reality through experimental validation. Direct observation of electron arrangement is, of course, impossible, but a range of sophisticated spectroscopic techniques provides irrefutable indirect evidence to support and refine our understanding of Chromium's unique electronic structure. These experimental findings are crucial in confirming the theoretical models that explain the anomaly.
Spectroscopic Techniques: Probing Electron Transitions
Spectroscopy, in its various forms, stands as a cornerstone in determining atomic electron configurations. The fundamental principle rests upon the interaction of electromagnetic radiation with matter.
Atoms absorb or emit photons of specific energies, corresponding to transitions between discrete electronic energy levels. By analyzing the wavelengths (or frequencies) of the absorbed or emitted radiation, valuable information can be extracted regarding the energy level spacing within the atom, ultimately revealing the electron configuration.
Atomic Emission Spectroscopy
Atomic Emission Spectroscopy (AES) involves exciting atoms to higher energy states, typically using heat or an electrical discharge. As the excited atoms return to their ground state, they emit photons of specific wavelengths.
The emitted light is then passed through a spectrometer, which separates the light into its constituent wavelengths, creating a spectrum. Each element possesses a unique emission spectrum, acting as a fingerprint that can be used to identify the element and, more importantly, infer its electron configuration.
The presence and intensity of specific spectral lines provide critical evidence for the presence of electrons in particular orbitals. Specifically, the experimentally observed emission spectrum of Chromium aligns with transitions predicted from the 4s1 3d5 configuration.
X-ray Spectroscopy
X-ray spectroscopy provides complementary information by probing the core electrons of an atom. When an atom is bombarded with X-rays, core electrons can be ejected, creating vacancies.
Electrons from higher energy levels then transition to fill these vacancies, emitting X-rays with energies characteristic of the element. By analyzing the energies and intensities of these emitted X-rays, it's possible to gain insights into the energy levels and populations of core electrons, corroborating information obtained from AES and providing a more complete picture of the electronic structure.
The fine structure observed in X-ray spectra is sensitive to the valence electron configuration. Precise measurements of these spectral features have confirmed the partially filled d-orbital character in Chromium.
Online Databases: Accessing and Interpreting Spectroscopic Data
The vast amount of spectroscopic data generated by these experimental techniques is meticulously compiled and made accessible through online databases. Among these, the NIST Atomic Spectra Database stands out as an invaluable resource for researchers and students alike.
The NIST Atomic Spectra Database
The NIST Atomic Spectra Database, maintained by the National Institute of Standards and Technology (NIST), provides a comprehensive compilation of atomic spectral data, including energy levels, wavelengths, and transition probabilities.
This database enables researchers to:
- Access experimentally determined energy levels for Chromium and its ions.
- Identify spectral lines associated with specific electronic transitions.
- Compare experimental data with theoretical calculations.
- Analyze isotopic shifts and hyperfine structure.
By consulting the NIST database, researchers can independently verify the experimental evidence supporting Chromium's electron configuration. This access provides a powerful tool for understanding and validating the unique electronic behavior of Chromium.
Access to reliable and experimentally validated data is critical for advancing our knowledge of atomic structure and chemical behavior. Databases like the NIST Atomic Spectra Database are indispensable tools for researchers and educators, ensuring the accuracy and accessibility of spectroscopic information. These databases empower the scientific community to explore and confirm the intricacies of electron configurations, like that of Chromium, driving further innovation.
Video: Chromium Electron Configuration: A Detailed Guide
FAQs
Why is chromium's electron configuration an exception to the Aufbau principle?
Chromium's expected electron configuration ([Ar] 4s² 3d⁴) is less stable than its actual configuration ([Ar] 4s¹ 3d⁵). This occurs because a half-filled d subshell provides extra stability due to increased exchange energy, resulting in a lower energy state. Therefore, one electron from the 4s orbital moves to the 3d orbital.
What is the correct chromium electron configuration?
The correct chromium electron configuration is [Ar] 4s¹ 3d⁵. This notation indicates that chromium has one electron in its 4s orbital and five electrons in its 3d orbitals. This is due to the increased stability of a half-filled d subshell.
How does Hund's rule apply to the 3d⁵ configuration in chromium?
Hund's rule states that electrons will individually occupy each orbital within a subshell before doubling up in any one orbital. The 3d⁵ configuration in the chromium electron configuration follows Hund's rule, with each of the five 3d orbitals containing one electron with parallel spin, maximizing stability.
How does the unusual chromium electron configuration affect its chemical properties?
The [Ar] 4s¹ 3d⁵ chromium electron configuration contributes to chromium's multiple oxidation states and its ability to form colored compounds. The loosely held 4s¹ electron readily participates in bonding, leading to diverse chemical behavior.
So, there you have it! Hopefully, this guide demystified the quirky world of chromium electron configuration and you now understand why it prefers to be a little different. Keep exploring the periodic table – there are plenty more exceptions and interesting electron arrangements out there to discover!