Retention Time: Chromatography Optimization Tips
In chromatographic separations, retention time serves as a critical identifier for analytes, reflecting the duration an analyte interacts with the stationary phase within the column; Agilent Technologies, a prominent vendor of chromatography systems, offers a range of columns and software tools that facilitate precise retention time measurements; column temperature, a key parameter in gas chromatography (GC) and high-performance liquid chromatography (HPLC), significantly influences analyte retention time; and method development chemists frequently adjust mobile phase composition to fine-tune retention time and achieve optimal separation, particularly when working with complex mixtures in pharmaceutical analysis or environmental monitoring.
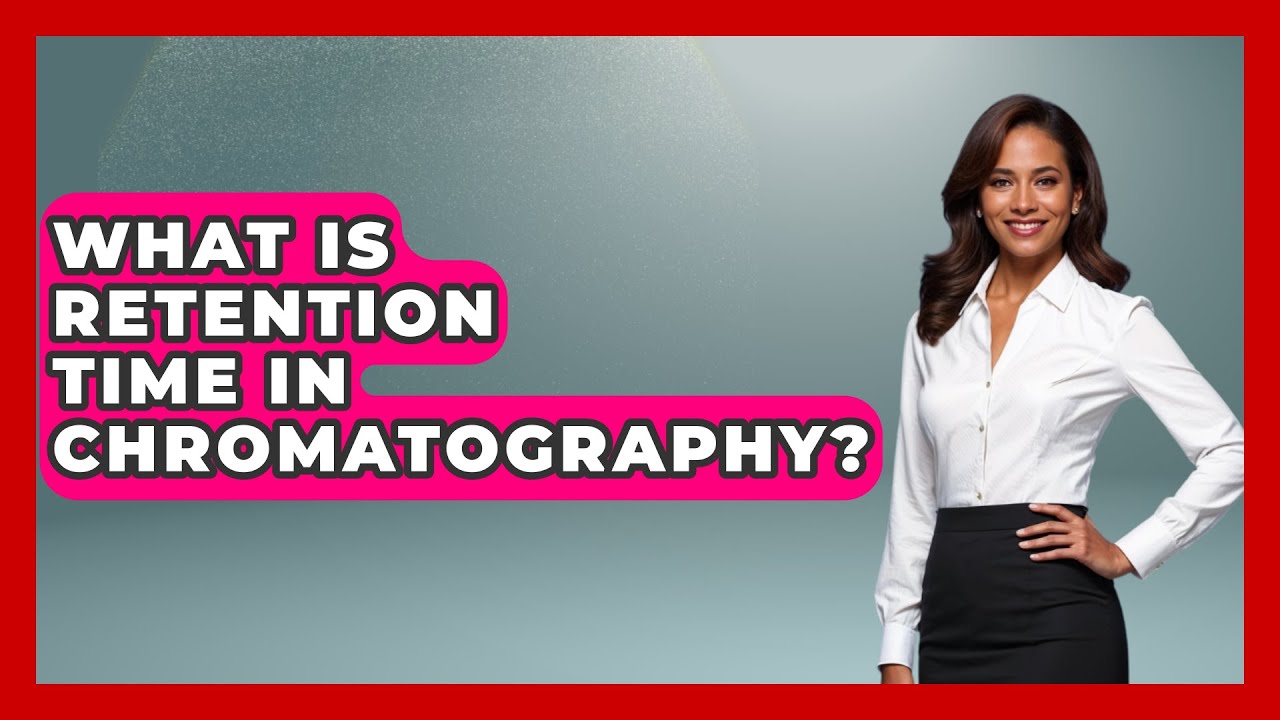
Image taken from the YouTube channel Chemistry For Everyone , from the video titled What Is Retention Time In Chromatography? - Chemistry For Everyone .
Chromatography stands as a cornerstone of modern analytical chemistry, a powerful technique employed to separate mixtures into their individual components. Its versatility and precision have made it indispensable across a wide range of scientific disciplines.
From ensuring the safety and efficacy of pharmaceuticals to monitoring environmental pollutants, chromatography plays a vital role in unraveling the complexities of chemical compositions. At its heart, chromatography leverages the differing physical and chemical properties of analytes.
The Essence of Chromatography: A Definition
Chromatography can be defined as a separation technique where components of a mixture are separated based on their differential interactions with two phases: a stationary phase and a mobile phase.
This interaction dictates the speed at which each component travels through the system, leading to their physical separation.
The Pervasive Importance of Chromatographic Analysis
The applications of chromatography are vast and varied, impacting numerous critical sectors.
-
Pharmaceutical Analysis: Ensuring drug purity, identifying metabolites, and monitoring drug stability.
-
Environmental Science: Detecting and quantifying pollutants in water, air, and soil.
-
Food Science: Analyzing food composition, identifying contaminants, and ensuring food safety.
-
Clinical Chemistry: Measuring levels of biomarkers in blood and urine for disease diagnosis.
-
Forensic Science: Identifying substances found at crime scenes for investigative purposes.
Mobile Phase: The Carrier Solvent
The mobile phase serves as the carrier, transporting the mixture through the chromatographic system.
It can be a liquid (in Liquid Chromatography, LC) or a gas (in Gas Chromatography, GC), chosen based on its ability to dissolve the sample and interact with the stationary phase. The choice of the mobile phase significantly impacts separation efficiency.
Stationary Phase: The Separation Medium
The stationary phase is a fixed material within the chromatographic system, responsible for interacting with the analytes and facilitating their separation.
It can be a solid (e.g., silica gel) or a liquid coated on a solid support. The nature of the stationary phase (e.g., polarity, particle size) determines which analytes are retained and to what extent. The interaction between the analytes and the stationary phase is crucial for effective separation.
Fundamental Principles: Understanding the Separation Mechanism
Chromatography stands as a cornerstone of modern analytical chemistry, a powerful technique employed to separate mixtures into their individual components. Its versatility and precision have made it indispensable across a wide range of scientific disciplines.
The magic of chromatography lies in the differential interaction of analytes with two distinct phases: the mobile phase and the stationary phase. This interaction dictates the separation process, with each analyte exhibiting a unique affinity for each phase. Understanding the underlying principles is crucial for method development and optimization.
Partitioning and Interactions Between Phases
At the heart of chromatography is the principle of partitioning. Analytes distribute themselves between the mobile and stationary phases based on their physical and chemical properties. This distribution is governed by the relative affinity of the analyte for each phase.
Analytes with a stronger affinity for the stationary phase will be retained longer, while those favoring the mobile phase will elute more quickly. This differential migration is what leads to separation. The nature of these interactions can vary widely, including:
-
Adsorption: Interaction based on surface attraction.
-
Partition: Dissolution of the analyte in the stationary phase.
-
Ion Exchange: Interaction based on ionic attraction.
-
Size Exclusion: Separation based on molecular size.
Key Parameters Affecting Separation Quality
Several parameters play a crucial role in influencing the chromatographic separation, each contributing to the overall efficiency and resolution of the separation process. Manipulating these factors allows for fine-tuning the separation to achieve optimal results.
Flow Rate
The flow rate of the mobile phase significantly impacts separation speed and efficiency. A higher flow rate reduces analysis time but can also compromise resolution by limiting the time available for analyte interaction with the stationary phase.
Conversely, a lower flow rate enhances resolution but increases analysis time. Optimization of flow rate is essential for balancing speed and resolution.
Temperature
Temperature is particularly relevant in Gas Chromatography (GC), where it directly influences the volatility of the analytes. Higher temperatures increase vapor pressure, promoting elution.
However, excessive temperatures can lead to analyte degradation. In Liquid Chromatography (LC), temperature can affect the viscosity of the mobile phase and the interactions between analytes and the stationary phase, although its impact is generally less pronounced than in GC.
pH
In Liquid Chromatography (LC), especially techniques like reversed-phase HPLC, pH plays a vital role by influencing the ionization state of acidic or basic analytes. The ionization state affects their hydrophobicity and, consequently, their retention on the stationary phase.
Adjusting the pH can selectively alter the retention of specific analytes, improving separation.
Void Volume (t0)
The void volume, often denoted as t0, represents the time it takes for an unretained compound to pass through the column. This parameter is critical for calculating the capacity factor (k’), which provides insights into the retention behavior of analytes.
Capacity Factor (k’)
The capacity factor (k’), also known as the retention factor, quantifies the retention of an analyte relative to the void volume. It is defined as:
k’ = (tR - t0) / t0
Where tR is the retention time of the analyte. A higher k’ value indicates stronger retention.
Optimizing k’ values is essential for achieving good separation, with values between 2 and 10 generally considered ideal.
Polarity
The polarity of both the mobile and stationary phases significantly influences analyte retention. In reversed-phase HPLC (RP-HPLC), a non-polar stationary phase is used in conjunction with a polar mobile phase.
Hydrophobic analytes are retained longer on the non-polar stationary phase, while polar analytes elute more quickly. The opposite is true for normal-phase chromatography.
Solvent Strength
Solvent strength refers to the eluting power of the mobile phase. In LC, a stronger solvent has a higher affinity for the stationary phase, effectively competing with the analytes and promoting their elution.
The choice of solvent and its concentration in the mobile phase can be adjusted to optimize separation. Gradient elution, where the solvent strength is gradually increased over time, is often used to separate complex mixtures.
The Elution Process and its Order
The elution process involves removing the separated analytes from the column for subsequent detection. The order in which analytes elute is determined by their relative affinities for the mobile and stationary phases.
Analytes with weaker interactions elute first, followed by those with stronger interactions. Understanding and controlling the elution order is crucial for accurate identification and quantification of the separated components. Optimizing the elution order can involve adjusting the mobile phase composition, temperature, or other relevant parameters to achieve the desired separation.
Performance Metrics: Evaluating Separation Quality
Chromatography stands as a cornerstone of modern analytical chemistry, a powerful technique employed to separate mixtures into their individual components. Its versatility and precision have made it indispensable across a wide range of scientific disciplines.
The magic of chromatography lies in the effective separation of analytes, but how do we objectively assess the quality of a chromatographic separation? Several key performance metrics provide a quantitative framework for evaluating separation efficacy, including efficiency, resolution, and selectivity. These parameters directly reflect the quality of the chromatographic process and its ability to distinguish between different compounds in a mixture.
Efficiency: Minimizing Peak Broadening
Chromatographic efficiency refers to the narrowness of the peaks obtained. High efficiency translates to sharp, well-defined peaks, indicating minimal band broadening during the separation process. Peak broadening is an undesirable phenomenon as it reduces resolution and makes accurate quantification difficult.
Several factors contribute to peak broadening. Diffusion is a natural process where analytes spread out as they move through the chromatographic system. Eddy diffusion occurs due to variations in flow paths within the column packing material, causing some molecules to travel shorter distances than others.
Careful column packing and optimized flow rates can help minimize these effects.
Understanding Peak Asymmetry: Tailing and Fronting
Ideally, chromatographic peaks should be symmetrical, resembling a Gaussian distribution. However, deviations from this ideal are common, leading to peak tailing or fronting.
Peak tailing, where the peak extends more gradually after the apex, is often caused by interactions between analytes and active sites on the stationary phase. These sites can temporarily retain analyte molecules, leading to a drawn-out elution.
Peak fronting, conversely, occurs when the peak rises more gradually than it declines. This can be caused by overloading the column, where the stationary phase becomes saturated with analyte.
Addressing peak asymmetry is crucial for accurate quantitative analysis.
Selectivity (α): The Power to Distinguish
Selectivity (α) quantifies the relative retention of two compounds in a chromatographic system. It's defined as the ratio of the retention factors (k') of two adjacent peaks.
A higher selectivity value indicates a greater difference in the affinity of the two compounds for the stationary phase. This results in a more significant separation between their peaks.
Selectivity is a critical factor in achieving good resolution. Without adequate selectivity, even highly efficient columns may fail to adequately separate closely related compounds. Optimizing mobile phase composition and stationary phase chemistry are key strategies to improve selectivity.
Resolution (Rs): The Ultimate Measure of Separation
Resolution (Rs) provides a comprehensive measure of the separation between two peaks, taking into account both their separation and their peak widths. It is the ultimate metric to determine whether two substances are separated enough.
Resolution is mathematically defined as:
Rs = 2(tR2 - tR1) / (w1 + w2)
Where:
- tR1 and tR2 are the retention times of the two peaks.
- w1 and w2 are the peak widths at the base.
A resolution value of 1.5 or greater is generally considered baseline resolution, meaning the peaks are sufficiently separated for accurate quantification. Achieving adequate resolution is the primary goal of chromatographic method development. It ensures that individual components can be accurately identified and quantified within a complex mixture.
Chromatographic Techniques: Exploring Diverse Methods
[Performance Metrics: Evaluating Separation Quality Chromatography stands as a cornerstone of modern analytical chemistry, a powerful technique employed to separate mixtures into their individual components. Its versatility and precision have made it indispensable across a wide range of scientific disciplines. The magic of chromatography lies in the...]
From the fundamental principles that govern separation to the metrics used to evaluate its success, we now turn our attention to the diverse array of chromatographic techniques. Each method leverages unique properties and is tailored for specific applications, making the selection of the appropriate technique critical for achieving optimal results. This section delves into the most common and powerful chromatographic techniques, highlighting their underlying principles and practical applications.
Gas Chromatography (GC)
Gas Chromatography (GC) is a workhorse technique in analytical chemistry, particularly suited for the analysis of volatile and thermally stable compounds. The fundamental principle behind GC is the separation of components based on their boiling points and interactions with a stationary phase.
The sample is first vaporized and then carried through a chromatographic column by an inert carrier gas, typically helium or nitrogen.
As the vaporized sample travels through the column, its components interact differently with the stationary phase, resulting in varying retention times. These differences in retention times enable the separation and subsequent detection of individual compounds.
Temperature Programming
One of the key advantages of GC is the ability to employ temperature programming. In this technique, the column temperature is gradually increased over time, which optimizes the separation of complex mixtures with a wide range of boiling points.
Starting at a lower temperature allows for the efficient separation of volatile compounds, while gradually increasing the temperature facilitates the elution of less volatile components.
Temperature programming significantly improves the resolution and sensitivity of GC analyses, making it an indispensable tool for many applications.
Liquid Chromatography (LC)
Liquid Chromatography (LC) serves as a complementary technique to GC, extending the reach of chromatography to non-volatile or thermally labile compounds that cannot withstand the high temperatures required in GC. In LC, a liquid mobile phase carries the sample through a column packed with a stationary phase.
The separation is based on the differential interactions of the sample components with the mobile and stationary phases.
High-Performance Liquid Chromatography (HPLC)
High-Performance Liquid Chromatography (HPLC) is a refined form of LC that employs high pressure to force the mobile phase through the column, resulting in faster separation times and improved resolution. HPLC is widely used in pharmaceuticals, environmental monitoring, and food science.
Reversed-Phase HPLC (RP-HPLC)
Reversed-Phase HPLC (RP-HPLC) is the most popular mode of HPLC, utilizing a non-polar stationary phase (typically a C18 or C8 bonded phase) and a polar mobile phase (such as water-methanol or water-acetonitrile mixtures). This technique is ideal for separating non-polar or moderately polar compounds.
The hydrophobic analytes are retained on the non-polar stationary phase, while the polar compounds elute more quickly.
Normal-Phase HPLC
Normal-Phase HPLC, conversely, employs a polar stationary phase (such as silica) and a non-polar mobile phase (such as hexane or ethyl acetate). This technique is suitable for separating polar compounds.
The polar analytes are retained on the polar stationary phase, while the non-polar compounds elute more quickly.
Ion Chromatography (IC)
Ion Chromatography (IC) is a specialized form of LC used for the separation and quantification of ions. It utilizes a stationary phase with ion-exchange properties and a mobile phase containing a buffer.
The ions in the sample are separated based on their charge and affinity for the ion-exchange resin. IC is widely used in environmental analysis for the determination of anions and cations in water samples, as well as in the food and beverage industry for quality control purposes.
Elution Methods
The method of elution, or how the mobile phase composition is managed during the chromatographic run, significantly affects separation. Two primary elution methods exist: isocratic and gradient elution.
Isocratic Elution
Isocratic elution involves using a constant mobile phase composition throughout the chromatographic run. While simple to implement, isocratic elution may not be suitable for separating complex mixtures with a wide range of polarities.
Gradient Elution
Gradient elution, on the other hand, involves changing the mobile phase composition over time. This technique is particularly useful for separating complex mixtures, as it allows for the selective elution of compounds based on their affinity for the mobile and stationary phases.
By gradually increasing the strength of the mobile phase, retained compounds can be eluted more efficiently, leading to sharper peaks and improved resolution. Gradient elution is a powerful tool for optimizing chromatographic separations.
Optimizing Column Performance: The Heart of Effective Chromatography
Chromatographic separation hinges on a complex interplay of factors, but the column itself, with its carefully chosen characteristics, forms the very heart of the process. Understanding and optimizing column parameters is paramount to achieving desired separation efficiency and resolution. Here, we delve into the critical operational parameters related to the chromatographic column, examining how column dimensions, particle size, and chemical modifications influence overall separation performance.
Column Dimensions: Length and Diameter
Column dimensions, specifically length and internal diameter, significantly impact both retention and backpressure. The column length directly affects the residence time of analytes within the stationary phase, thereby influencing separation.
Longer columns generally provide greater resolution as they increase the opportunity for interaction between the analytes and the stationary phase. This leads to improved separation of complex mixtures, but at the cost of increased analysis time and higher backpressure.
Shorter columns, conversely, offer faster analysis times and lower backpressure. However, they may not provide sufficient resolution for complex mixtures.
The internal diameter of the column also plays a crucial role. Narrow-bore columns offer increased sensitivity due to reduced analyte dilution, making them suitable for analyzing trace amounts of substances.
Wider-bore columns, on the other hand, can handle larger sample volumes and are less susceptible to clogging. However, they may exhibit lower sensitivity. The choice of column diameter depends largely on the sample complexity, concentration, and detection method employed.
Stationary Phase Particle Size: Balancing Efficiency and Pressure
The particle size of the stationary phase material is a critical determinant of column efficiency and backpressure. Smaller particle sizes generally lead to increased column efficiency due to a reduction in band broadening. This translates to sharper peaks and improved resolution.
However, reducing particle size also results in a dramatic increase in backpressure. This heightened pressure places greater demands on the chromatographic system.
Ultra-high-performance liquid chromatography (UHPLC) utilizes columns packed with sub-2 μm particles to achieve exceptionally high resolution and speed. The price is significantly higher backpressure.
Conventional HPLC typically employs columns with larger particle sizes (e.g., 3-5 μm) to maintain manageable backpressures. Selecting the appropriate particle size involves a delicate balance between the desired efficiency and the system's pressure capabilities.
Chemical Modification of the Stationary Phase: Tailoring Selectivity
The chemical modification of the stationary phase allows for tailoring its selectivity to target specific types of analytes. This is achieved by bonding different functional groups to the surface of the support material, creating a variety of stationary phases with distinct properties.
For reversed-phase chromatography, octadecylsilane (C18) and octylsilane (C8) are commonly used stationary phases. C18 phases are highly hydrophobic and provide strong retention for nonpolar compounds.
C8 phases, having shorter alkyl chains, exhibit weaker retention and are useful for separating more polar compounds or for reducing analysis time. In normal-phase chromatography, bare silica is used as a polar stationary phase.
Chemical modification enables chromatographers to fine-tune the separation process, optimizing the resolution and selectivity for specific applications. By understanding and manipulating these operational parameters, analysts can unlock the full potential of chromatographic techniques. This leads to more accurate, efficient, and reliable analytical results.
Troubleshooting: Addressing Common Issues in Chromatography
Optimizing column performance is crucial, but even with the best setup, chromatographic systems can encounter issues that compromise data quality and analytical accuracy. Effectively addressing these problems requires a systematic approach, combining a thorough understanding of chromatographic principles with diligent observation and problem-solving skills. This section delves into common chromatographic challenges, offering practical guidance to troubleshoot and maintain optimal system performance.
Identifying and Addressing Common Chromatographic Problems
Chromatographic troubleshooting isn't about guesswork; it's a methodical process of identifying the root cause of observed issues and applying targeted solutions. We will explore strategies for mitigating drifting retention times, reducing baseline noise, and correcting distorted peak shapes.
Drifting Retention Times: Diagnosing and Resolving Instability
Retention time drift, characterized by gradual shifts in analyte retention over time, can significantly impact the reliability of quantitative analysis. Causes can range from variations in mobile phase composition and flow rate to temperature fluctuations or column degradation.
-
System Instability: Ensure stable temperature control of the column and autosampler. Regularly check and calibrate pumps to maintain consistent flow rates.
-
Mobile Phase Variations: Prepare fresh mobile phase solutions using high-purity solvents. Ensure proper degassing to prevent air bubbles. For gradient elution, verify the accuracy of solvent mixing ratios.
-
Column Degradation: Over time, the stationary phase can degrade, leading to altered retention characteristics. Consider column replacement or regeneration, if appropriate for the column type. Gradual loss of bonded phase from silica-based columns is a common cause.
-
Injection Volume Variations: Ensure accurate injection volumes by calibrating the autosampler and inspecting the syringe and injector for leaks or blockages.
Mitigating Baseline Noise: Strategies for a Quieter Signal
Excessive baseline noise obscures analyte peaks, reducing sensitivity and hindering accurate quantification. The noise can originate from various sources, requiring a systematic approach to identify and eliminate the contributing factors.
-
Detector Issues: Check the detector lamp intensity and alignment. High detector gains can amplify baseline noise, and should be set as low as possible while maintaining acceptable signal.
-
Electrical Noise: Ensure proper grounding of all instruments to minimize electrical interference. Separate power lines for sensitive equipment.
-
Solvent Contamination: Use high-purity solvents and filter the mobile phase to remove particulate matter. Even trace impurities can contribute to baseline noise.
-
Air Bubbles: Thoroughly degas the mobile phase to prevent air bubbles from forming in the flow path, especially within the detector cell. An in-line degasser is highly recommended.
-
Temperature Fluctuations: Ensure consistent temperature in the laboratory environment to prevent variations in refractive index or solvent viscosity, which can contribute to baseline fluctuations.
Resolving Peak Shape Issues: Dealing with Tailing, Fronting, and Broadening
Non-ideal peak shapes, such as tailing, fronting, or excessive broadening, can degrade resolution and compromise quantitative accuracy. Addressing these issues requires understanding the underlying mechanisms that cause these distortions.
Peak Tailing
Peak tailing often arises from secondary interactions between the analyte and the stationary phase, such as silanol groups in silica-based columns.
- Silanol Activity: In reversed-phase HPLC, tailing can be reduced by using end-capped columns, which minimize silanol interactions. Add a small amount of a competing base, such as triethylamine (TEA), to the mobile phase, but TEA can be detrimental to mass spectrometry.
- Column Overload: Ensure that the injected amount of analyte is within the linear range of the detector. Overloading the column can lead to peak distortion.
- Sample Matrix Effects: Matrix components can interfere with analyte retention. Sample cleanup or matrix-matched calibration can mitigate these effects.
Peak Fronting
Peak fronting, where the leading edge of the peak is steeper than the trailing edge, is less common but can occur due to column overload or injection solvent effects.
- Column Overload: As with tailing, reduce the amount of analyte injected.
- Solvent Mismatch: Ensure that the injection solvent is weaker than the mobile phase to prevent band broadening and fronting.
- Dead Volume: Minimize dead volume in the system to prevent band spreading.
Peak Broadening
Peak broadening reduces resolution and sensitivity. It can arise from various factors affecting the efficiency of the separation process.
- Extra-Column Volume: Minimize extra-column volume by using short, narrow connecting tubing and minimizing detector cell volume.
- Flow Rate: Optimizing the flow rate can reduce band broadening. Too low of a flowrate allows too much time for diffusion of the analytes. Too high of a flowrate can cause the column to be inefficient.
- Column Temperature: In GC, proper temperature programming can minimize peak broadening. In LC, consistent column temperature ensures reproducible retention.
- Particle Size: Smaller particle sizes in the stationary phase generally lead to narrower peaks and improved resolution, but at the expense of higher backpressure.
By systematically addressing these common issues, chromatographers can maintain system performance, ensure data quality, and maximize the accuracy and reliability of their analyses.
Video: Retention Time: Chromatography Optimization Tips
FAQs: Retention Time & Chromatography Optimization
What exactly is retention time in chromatography?
Retention time is the time it takes for a specific analyte to pass through the chromatographic system, from injection to detection. It's a key identifier for compounds and is influenced by the compound's interaction with both the stationary and mobile phases.
How does column temperature affect retention time?
Generally, increasing the column temperature decreases retention time in both gas chromatography (GC) and liquid chromatography (LC). Higher temperatures improve analyte volatility in GC and alter solvent viscosity and analyte interaction in LC, leading to faster elution.
What mobile phase adjustments can I make to optimize retention time?
In reversed-phase LC, increasing the organic solvent concentration in the mobile phase usually decreases the retention time. In normal-phase LC, increasing the polarity of the mobile phase typically decreases retention time.
My compound isn't eluting. How can I decrease its retention time?
To decrease retention time, you can try increasing the column temperature, altering the mobile phase composition to be more eluting (e.g., more organic solvent in reversed-phase), or using a different stationary phase with a different selectivity. Selecting a different column with a weaker retention character might also help.
So, there you have it! A few tips and tricks to help you wrangle that retention time and get the separation you need. Chromatography can be finicky, but with a little experimentation and these adjustments, you'll be well on your way to optimizing your method and getting consistent, reliable results. Happy analyzing!