Carbon: Protons, Neutrons, Electrons Explained
Carbon, a cornerstone of organic chemistry, owes its unique properties to the arrangement of its subatomic particles. The nucleus of a carbon atom, investigated extensively by researchers at institutions like the Lawrence Berkeley National Laboratory, contains six protons, defining its atomic number. In addition to protons, the carbon nucleus also includes neutrons; the quantity of these neutrons can vary, leading to different isotopes, a concept further elucidated by the work of scientists such as Marie Curie. Orbiting this nucleus are six electrons, crucial for carbon's ability to form diverse chemical bonds, a phenomenon modeled and visualized using tools like computational software Gaussian. Understanding the configuration of carbon protons neutrons electrons is fundamental to grasping the behavior of carbon-based compounds, which are essential components of life and materials around us.
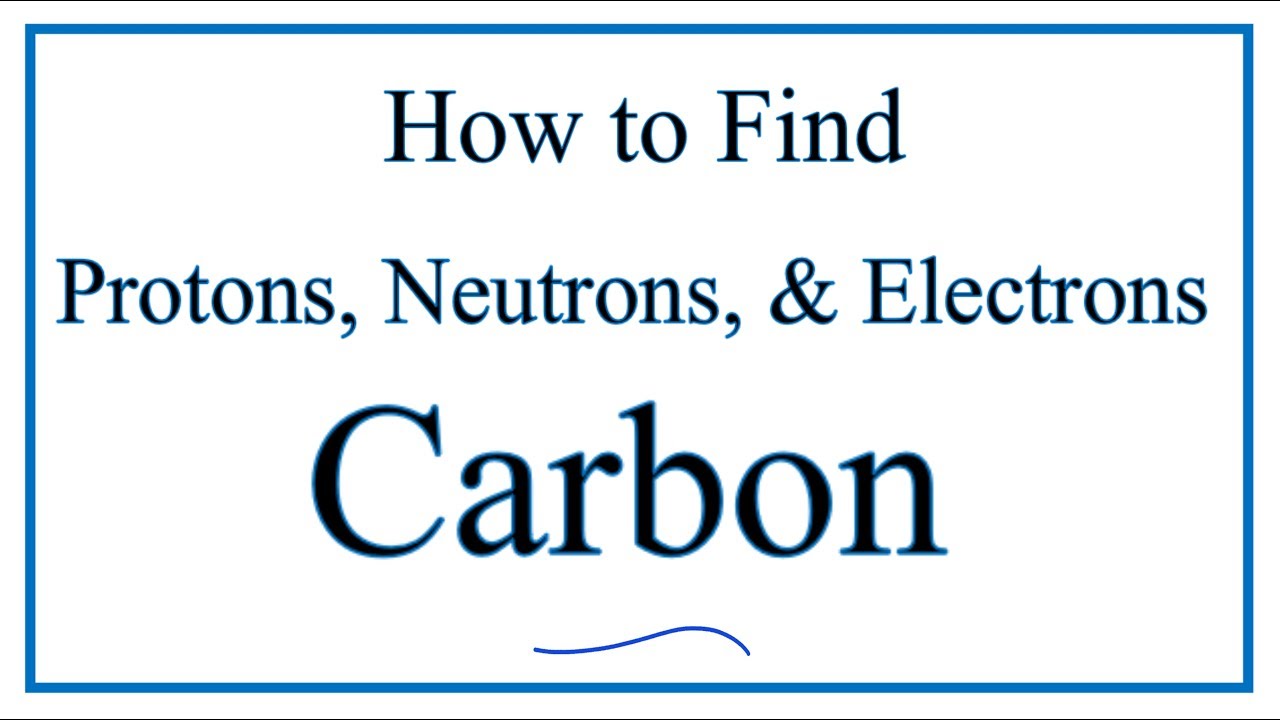
Image taken from the YouTube channel Wayne Breslyn (Dr. B.) , from the video titled How to find the Number of Protons, Electrons, Neutrons for Carbon (C) .
Unveiling the Building Blocks of Matter: A Journey into Atomic Structure
Ever wondered what everything around us is really made of? The answer, in its most basic form, lies in the realm of atoms – the fundamental building blocks of matter. Understanding atomic structure is like possessing a secret key, unlocking insights into how materials behave, how chemical reactions occur, and ultimately, how the world works.
But simply knowing atoms exist isn't enough. We need to understand how they are built, and that's where the real fun begins.
Atoms: More Than Just Tiny Spheres
The classic image of an atom as a miniature solar system, with electrons orbiting a nucleus, is a good starting point. But the reality is far more nuanced, and frankly, a bit mind-bending. This is where quantum mechanics enters the picture.
Quantum mechanics is a branch of physics that governs the behavior of matter at the atomic and subatomic levels. It challenges our everyday intuition, introducing concepts like wave-particle duality and quantized energy levels. Instead of thinking of electrons as orbiting in neat paths, quantum mechanics paints a picture of probability clouds, representing the likelihood of finding an electron in a particular region of space.
Think of it like this: we can’t know exactly where an electron is at any given moment, but we can define areas where it probably is.
Why Should You Care About Atomic Structure?
So, why is all of this important? Because understanding atomic structure and quantum mechanics is essential for a wide range of fields.
In chemistry, it allows us to predict how atoms will interact to form molecules and compounds.
In materials science, it helps us design new materials with specific properties, like strength, conductivity, or reactivity.
In medicine, it enables the development of new drugs and therapies that target specific molecules in the body.
The implications stretch even further – from designing more efficient solar panels to understanding the origins of the universe. It's a foundational understanding that empowers innovation.
Your Roadmap to Understanding
In this journey, we'll start with the basics: protons, neutrons, and electrons, and how they define an atom. Then, we'll dive into the quantum mechanical model, exploring the world of quantum numbers and probability distributions.
Next, we'll acknowledge the brilliant minds who paved the way, celebrating the scientists who unveiled the secrets of the atom.
Finally, we'll touch upon how these fundamental building blocks join forces to form the bonds that hold our world together.
Buckle up – it’s going to be an atomic ride!
Foundations of Atomic Structure: Protons, Neutrons, and Electrons
Unveiling the secrets of matter requires a solid understanding of its fundamental components. Let's embark on a journey to explore the very core of atoms, starting with the particles that define their identity and behavior: protons, neutrons, and electrons. This section will lay the groundwork, defining key terms and concepts that are essential for grasping more complex aspects of atomic theory.
The Nucleus and Its Components
At the heart of every atom lies the nucleus, a dense region containing positively charged protons and neutral neutrons. These particles are the heavyweights of the atomic world, contributing significantly to an atom's mass. But how do we differentiate between elements and their variations?
Atomic Number
The atomic number is the single most important characteristic of an element. It represents the number of protons found in the nucleus of an atom of that element.
For instance, every atom with one proton is hydrogen, and every atom with six protons is carbon.
The atomic number is like an element's unique ID, defining its identity and distinguishing it from all others. It's the cornerstone of the periodic table.
Mass Number
The mass number, on the other hand, tells us the total number of protons and neutrons in an atom's nucleus. It gives us an approximate idea of the atom's mass.
To find the number of neutrons, simply subtract the atomic number (number of protons) from the mass number.
However, it's crucial to remember that the mass number is not the same as the atomic mass, which is a weighted average considering all the isotopes of an element.
Isotopes
Now, here’s where things get a little more interesting. Atoms of the same element can have different numbers of neutrons.
These variations are called isotopes.
For example, carbon-12 (12C) has 6 protons and 6 neutrons, while carbon-14 (14C) has 6 protons and 8 neutrons. Both are carbon, but their masses differ.
Isotopes play a significant role in various applications, from carbon dating in archaeology to medical imaging.
Electron Arrangement
While the nucleus dictates an element's identity and mass, the electrons, those tiny negatively charged particles whizzing around the nucleus, determine how an atom interacts with other atoms. Their arrangement is key to understanding chemical bonding.
Electron Configuration
Electrons are not randomly scattered around the nucleus; they occupy specific energy levels and orbitals. The electron configuration describes exactly how these electrons are distributed.
Understanding electron configuration allows us to predict an atom's chemical behavior.
Valence Electrons
Among all the electrons, the valence electrons hold a special significance. These are the electrons located in the outermost energy level of an atom.
They are the ones involved in forming chemical bonds with other atoms.
The number of valence electrons determines an atom's reactivity and the types of bonds it can form. An atom with a full valence shell is stable and unreactive, while an atom with an incomplete valence shell will readily bond with other atoms to achieve stability.
Quantum Mechanical Model: A Probabilistic View of the Atom
Having grasped the foundational elements of atomic structure, we now venture into the realm of quantum mechanics.
This isn't your classical physics anymore; prepare to embrace a world where probabilities reign supreme, and electrons are less like tiny planets orbiting a sun and more like fuzzy clouds of potential.
The quantum mechanical model provides a more accurate, albeit less intuitive, picture of the atom. Let's dive in!
Quantum Theory Basics
At the heart of modern atomic understanding lies quantum theory. It's a shift from thinking of electrons as particles with defined paths to viewing them as existing in a state of probability.
Core Ideas of Quantum Mechanics
Quantum mechanics, in essence, tells us that the behavior of matter at the atomic and subatomic level is governed by probabilities, not certainties.
Think of it like this: instead of knowing exactly where an electron is at any given moment, we can only describe the probability of finding it in a particular region of space.
This probability is described by a mathematical function called a wave function.
Quantum mechanics introduces the concept of quantization, meaning that energy, momentum, and other properties can only take on specific, discrete values.
It's like climbing a staircase rather than a ramp; you can only stand on specific steps, not in between.
Demystifying Quantum Numbers
Quantum numbers are like the address of an electron within an atom. They provide a set of values that describe its properties, such as energy, shape, and spatial orientation.
There are four main quantum numbers:
-
Principal Quantum Number (n): This number describes the energy level of the electron. It can be any positive integer (n = 1, 2, 3, ...), with higher numbers indicating higher energy levels. Think of it as the "shell" the electron occupies.
-
Angular Momentum or Azimuthal Quantum Number (l): This number describes the shape of the electron's orbital and has values ranging from 0 to n-1. l = 0 corresponds to an s orbital (spherical), l = 1 corresponds to a p orbital (dumbbell-shaped), l = 2 corresponds to a d orbital (more complex shapes), and so on.
-
Magnetic Quantum Number (ml): This number describes the orientation of the electron's orbital in space. It can take on integer values from -l to +l, including 0. For example, if l = 1 (a p orbital), ml can be -1, 0, or +1, corresponding to the three different p orbitals oriented along the x, y, and z axes.
-
Spin Quantum Number (ms): This number describes the intrinsic angular momentum of the electron, which is also quantized and is called spin. Electrons behave as if they are spinning, creating a magnetic dipole moment. This spin can be either spin up (+1/2) or spin down (-1/2).
Principles and Rules Governing Electron Behavior
With an understanding of the quantum numbers, we now need to understand the rules governing how electrons populate the available orbitals within an atom. Two crucial principles come into play: the Pauli Exclusion Principle and Hund's Rule.
Pauli Exclusion Principle
The Pauli Exclusion Principle states that no two electrons in an atom can have the same set of all four quantum numbers.
This seemingly simple rule has profound consequences.
It dictates that each orbital can hold a maximum of two electrons, and these two electrons must have opposite spins (+1/2 and -1/2).
This principle is the foundation for understanding the electron configuration of atoms and the periodic table's structure.
Hund's Rule
Hund's Rule provides guidance on how electrons fill orbitals within a subshell (orbitals with the same n and l values, like the three p orbitals).
It states that electrons will individually occupy each orbital within a subshell before doubling up in any one orbital.
Moreover, these single electrons will all have the same spin.
This maximizes the overall spin and minimizes the energy of the atom. Think of it like this: electrons prefer to have their own space and spin in the same direction before pairing up.
This minimizes electron-electron repulsion and leads to a more stable configuration.
Key Figures in Atomic Theory: Pioneers of Discovery
Having navigated the abstract landscape of quantum mechanics, it's time to anchor ourselves in the real world by acknowledging the brilliant minds who paved the way.
These weren't just lab-coat-clad figures scribbling equations; they were visionaries who dared to challenge existing paradigms and, in doing so, revolutionized our understanding of the universe. Let's meet some of the key players:
Early Pioneers: Unveiling the Atom's Secrets
Ernest Rutherford: The Gold Foil Revelation
Ernest Rutherford's name is synonymous with the discovery of the atomic nucleus.
His famous gold foil experiment, conducted in 1909, was a watershed moment.
By firing alpha particles at a thin gold foil, Rutherford observed that while most particles passed straight through, a small fraction were deflected at large angles.
This led him to conclude that the atom's positive charge and most of its mass were concentrated in a tiny, dense core – the nucleus.
Rutherford’s model, though not perfect, demolished the previous "plum pudding" model and set the stage for future advancements.
Niels Bohr: Quantized Energy Levels
Building upon Rutherford's work, Niels Bohr introduced the concept of quantized energy levels.
Bohr proposed that electrons could only orbit the nucleus at specific, discrete energy levels, like rungs on a ladder.
This was a radical departure from classical physics, which allowed for continuous energy values.
When an electron jumps from one energy level to another, it absorbs or emits energy in the form of light.
This explained the discrete spectral lines observed in atomic emissions and provided a framework for understanding atomic behavior.
Bohr's model wasn’t without its limitations, but it laid the groundwork for quantum mechanics.
Quantum Mechanics Contributors: Embracing Uncertainty
Werner Heisenberg: The Uncertainty Principle
Werner Heisenberg is best known for his formulation of the uncertainty principle.
This principle states that it is impossible to know both the position and momentum of a particle with perfect accuracy.
The more precisely we know one, the less precisely we know the other.
This isn't a limitation of our measuring instruments; it's a fundamental property of the universe.
The uncertainty principle shook the foundations of classical determinism and forced scientists to embrace a probabilistic view of reality.
Heisenberg's work helped shape our understanding of the inherent limitations in observing and predicting the behavior of quantum systems.
Erwin Schrödinger: The Wave Equation
Erwin Schrödinger developed the Schrödinger equation, a mathematical equation that describes the behavior of quantum mechanical systems.
This equation allows us to calculate the probability of finding an electron in a particular region of space.
The Schrödinger equation is a cornerstone of quantum mechanics, providing a powerful tool for understanding the behavior of atoms and molecules.
It gives rise to the concept of atomic orbitals, which are three-dimensional regions around the nucleus where electrons are most likely to be found.
Discoveries of Subatomic Particles: Completing the Picture
James Chadwick: Unmasking the Neutron
James Chadwick's discovery of the neutron in 1932 completed the picture of the atom's fundamental constituents.
Before Chadwick, scientists knew about protons and electrons, but the mass of the nucleus couldn't be explained by protons alone.
Chadwick demonstrated the existence of a neutral particle, the neutron, with a mass similar to that of the proton.
This discovery resolved the mass discrepancy and provided a more complete understanding of nuclear structure.
The discovery of the neutron opened up new avenues of research in nuclear physics and paved the way for the development of nuclear technology.
Chemical Bonding: Atoms Joining Forces
Having explored the intricate world of individual atoms and their quantum quirks, it's time to see how these tiny building blocks come together to form the stuff around us. Chemical bonding is the glue that holds matter together, and understanding it is key to understanding everything from the properties of water to the complexity of life itself.
We're going to focus on covalent bonding as our primary example because it beautifully illustrates the fundamental principle of atoms striving for stability. But before we dive in, let's set the stage with a general overview of why atoms even bother to bond in the first place.
The Octet Rule: Why Atoms Bond
Atoms, like people, crave stability. For atoms, stability often means having a full outer shell of electrons—specifically, eight electrons, hence the "octet rule." Noble gases like neon and argon already have this magic number, which is why they are chemically inert. They are complete and don't need to react with anyone.
Other elements, however, are not so lucky. They need to gain, lose, or share electrons to achieve this stable configuration. This is where chemical bonding comes into play. When atoms interact, they are trying to achieve this octet.
Covalent Bonding: Sharing is Caring
Unlike ionic bonds where electrons are transferred between atoms, covalent bonds involve the sharing of electrons. This sharing typically occurs between two nonmetal atoms. Instead of one atom completely giving up an electron to another, they decide to cooperate, sharing a pair of electrons between them.
The Formation of a Covalent Bond
Imagine two hydrogen atoms approaching each other. Each hydrogen atom has only one electron and yearns for a second to fill its outermost shell (in this case, a duet is needed, not an octet). As they get closer, their electron clouds start to overlap. The shared electron pair now spends most of its time in the space between the two nuclei, effectively holding them together. This shared electron pair constitutes a covalent bond.
This bond creates a new entity, a molecule of hydrogen gas (H₂), which is more stable than two separate hydrogen atoms. Now, both hydrogens feel like they have two electrons at least some of the time.
Single, Double, and Triple Bonds
Atoms can share more than one pair of electrons. If two atoms share one pair, it's a single bond. Share two pairs, it's a double bond. And if they share three pairs, it's a triple bond. As you might expect, the more electron pairs shared, the stronger and shorter the bond tends to be.
Think of nitrogen gas (N₂). Each nitrogen atom needs three more electrons to complete its octet. They achieve this by forming a triple bond, sharing six electrons (three pairs) between them. This strong triple bond is why nitrogen gas is relatively inert and makes up a large portion of our atmosphere.
Polar Covalent Bonds and Electronegativity
Not all covalent bonds are created equal. In some cases, one atom might be greedier than the other, pulling the shared electrons closer to itself. This "greediness" is known as electronegativity.
If there's a significant difference in electronegativity between the two atoms, the shared electrons will spend more time around the more electronegative atom, creating a polar covalent bond. This results in a partial negative charge (δ-) on the more electronegative atom and a partial positive charge (δ+) on the less electronegative atom.
Water (H₂O) is a classic example. Oxygen is much more electronegative than hydrogen, so the oxygen atom carries a partial negative charge, while the hydrogen atoms carry partial positive charges. This polarity is responsible for many of water's unique properties, such as its ability to dissolve a wide range of substances.
Video: Carbon: Protons, Neutrons, Electrons Explained
FAQs: Carbon: Protons, Neutrons, Electrons Explained
What determines that an atom is carbon?
The number of protons in its nucleus. All carbon atoms have exactly 6 protons. This is what defines an element. The number of neutrons and electrons can vary, but if it has 6 protons, it's carbon. Carbon protons neutrons electrons define its very essence.
How can carbon atoms have different weights?
Carbon atoms can have different numbers of neutrons in their nucleus. Atoms of the same element with different neutron counts are called isotopes. While the number of carbon protons neutrons electrons count will be the same, the number of neutrons will vary.
What role do electrons play in carbon's behavior?
Electrons are responsible for how carbon interacts with other atoms, forming chemical bonds. Carbon has 6 electrons, and their arrangement allows it to form a wide variety of bonds, making it essential to life. Carbon protons neutrons electrons all contribute to the structure of an atom.
Why is carbon so important in organic chemistry?
Carbon has a unique ability to form stable chains and rings with itself. This, coupled with its capacity to bond with hydrogen, oxygen, nitrogen, and other elements, allows for the vast diversity of organic molecules. Carbon protons neutrons electrons all play a vital role in carbon's bonding.
So, next time you see "C" on the periodic table, remember it's not just a letter! Think about all the amazing things carbon protons neutrons electrons make possible, from the graphite in your pencil to the very building blocks of life. Pretty cool, right?