Calvin Cycle Outputs: What Plants Produce
Photosynthesis in plants encompasses a complex series of biochemical reactions, and within this process, the Calvin cycle plays a critical role in carbon fixation, with its outputs being crucial for plant growth. Specifically, the Calvin cycle outputs include glyceraldehyde-3-phosphate (G3P), a three-carbon sugar that serves as the precursor for glucose and other carbohydrates; this process is facilitated by the enzyme RuBisCO, which catalyzes the initial carbon fixation step. These carbohydrates produced are then utilized by plants as a source of energy for cellular respiration and as building blocks for structural components like cellulose, which forms the cell walls, while the efficiency of this cycle can be studied at institutions like the Carnegie Institution for Science, where many research projects focus on plant biology. In essence, the Calvin cycle's ability to generate G3P determines a plant's capacity to synthesize essential organic molecules needed to sustain life.
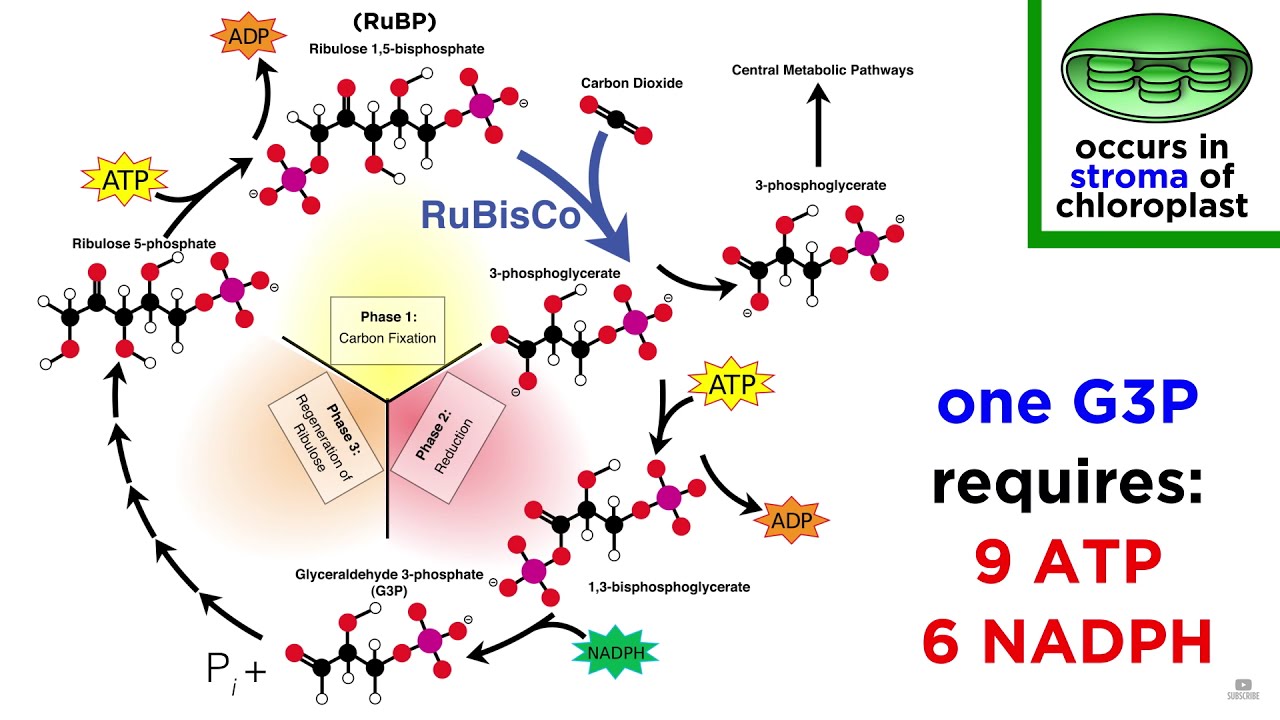
Image taken from the YouTube channel Professor Dave Explains , from the video titled Photosynthesis: Light Reactions and the Calvin Cycle .
Demystifying the Calvin Cycle: The Engine of Sugar Production
Photosynthesis, the remarkable process fueling nearly all life on Earth, hinges on converting light energy into chemical energy, effectively producing sugars from carbon dioxide and water.
This fundamental process unfolds in two key stages: the light-dependent reactions and the Calvin Cycle. While the light-dependent reactions capture sunlight and transform it into chemical energy in the form of ATP and NADPH, it is the Calvin Cycle that harnesses this energy to drive the fixation of carbon dioxide into sugars.
The Calvin Cycle, sometimes referred to as the "dark reactions" or "light-independent reactions" (though these terms can be misleading as the cycle is indirectly dependent on light), represents the linchpin for carbon assimilation in plants.
The Calvin Cycle: Carbon Fixation and Sugar Synthesis
At its core, the Calvin Cycle is a series of biochemical reactions that fix atmospheric carbon dioxide (CO2), incorporating it into an existing organic molecule, Ribulose-1,5-bisphosphate (RuBP).
This initial fixation is catalyzed by the enzyme RuBisCO, one of the most abundant proteins on Earth.
The subsequent reactions, driven by the ATP and NADPH generated during the light-dependent reactions, reduce the fixed carbon to produce glyceraldehyde-3-phosphate (G3P), a three-carbon sugar.
G3P serves as the precursor for glucose and other complex sugars, the very foundation of plant biomass and the primary source of energy for many organisms.
The Stroma: The Calvin Cycle's Operational Base
The Calvin Cycle operates within the stroma of the chloroplast, the organelle responsible for photosynthesis in plant cells.
The stroma provides the necessary enzymatic machinery and optimal conditions for the complex series of reactions to occur.
This location is critical, as it allows the Calvin Cycle to directly utilize the ATP and NADPH produced by the light-dependent reactions, which occur within the thylakoid membranes also located inside the chloroplast.
This spatial proximity ensures efficient energy transfer and maximizes the overall efficiency of photosynthesis.
Pioneers of Photosynthesis: Unveiling the Calvin Cycle's Secrets
The intricate dance of carbon fixation within the Calvin Cycle owes its revelation to the tireless efforts of dedicated scientists. Their work, often collaborative, built upon existing knowledge, gradually illuminating the pathways of sugar synthesis in plants. This section recognizes the key figures whose contributions were indispensable in deciphering this vital process.
Melvin Calvin: The Architect of the Cycle
Melvin Calvin, a name synonymous with the cycle itself, spearheaded the groundbreaking research that unveiled the Calvin Cycle's mechanisms. His meticulous work, primarily conducted at the University of California, Berkeley, involved innovative use of radioactive carbon-14 as a tracer.
By exposing algae to carbon dioxide labeled with carbon-14 and interrupting the process at various intervals, Calvin and his team could identify the sequence in which different organic compounds were formed.
This painstaking approach allowed them to map the cyclical pathway of carbon fixation. Calvin's outstanding contribution was recognized in 1961 with the Nobel Prize in Chemistry. His legacy extends beyond the specific details of the cycle to exemplify the power of isotopic tracing in biochemical research.
Andrew Benson: Illuminating the Pathway
While Calvin's name is most widely associated with the cycle, Andrew Benson played a critical, yet often understated, role in its discovery.
Benson's expertise in analytical chemistry proved invaluable in identifying and characterizing the various intermediate compounds formed during carbon fixation. He was instrumental in elucidating the sequence of reactions and the enzymes involved.
His collaborative partnership with Calvin was crucial in piecing together the complete picture of the Calvin Cycle. Benson’s work, deeply intertwined with Calvin's, underscores the frequently collaborative nature of scientific discovery, where different expertise contributes to major breakthroughs.
James Bassham: Mapping the Metabolic Landscape
James Bassham further contributed to the unraveling of the Calvin Cycle by meticulously identifying and characterizing the various intermediate compounds involved.
His work provided critical details about the precise chemical transformations occurring at each step of the cycle. Bassham's contributions were essential in constructing the comprehensive metabolic map of carbon fixation.
His work helped solidify the understanding of the cyclical nature of the process. His dedication provided a crucial layer of detail to the overarching framework established by Calvin and Benson.
Carbon Fixation: Capturing Atmospheric CO2
The Calvin Cycle's operation hinges on an initial and vital step: carbon fixation. This process transforms inorganic carbon, sourced directly from atmospheric carbon dioxide, into an organic form that plants can utilize. This transition marks the very beginning of sugar synthesis and fuels the entire biosphere.
The Significance of Carbon Fixation
Carbon fixation is not merely an entry point; it's the linchpin connecting the abiotic (non-living) world to the biotic (living) one. Without it, carbon, a fundamental building block of all organic molecules, would remain locked in the atmosphere, unavailable for incorporation into plant tissues and, subsequently, the food chain.
Through carbon fixation, carbon dioxide is effectively "captured" and converted into a usable form, enabling the creation of more complex organic molecules like glucose and other sugars. This act is foundational to life on Earth.
RuBP: The Initial Carbon Dioxide Acceptor
The molecule responsible for initially grabbing carbon dioxide is ribulose-1,5-bisphosphate, or RuBP. This five-carbon sugar acts as the cycle's primary CO2 acceptor. It is already present within the stroma of the chloroplast, awaiting the arrival of carbon dioxide.
The structure of RuBP is key to its role; its molecular shape and chemical properties allow it to readily bind with CO2. It’s like the perfect molecular "docking station" for carbon dioxide.
RuBisCO: The Catalytic Engine of Carbon Fixation
The enzyme that drives the carbon fixation reaction is ribulose-1,5-bisphosphate carboxylase/oxygenase, commonly known as RuBisCO. This enzyme is arguably the most abundant protein on Earth, a testament to its critical function in photosynthesis.
RuBisCO’s role is to catalyze the carboxylation of RuBP. In simpler terms, it helps RuBP grab onto the incoming carbon dioxide molecule, initiating the next phase of the cycle. Without RuBisCO, the reaction would proceed far too slowly to sustain life.
The Unstable Intermediate and 3-PGA Formation
The immediate product of RuBisCO's action, the combination of RuBP and CO2, is an unstable six-carbon compound. This molecule is so unstable that it almost instantly breaks down.
This breakdown yields two molecules of a three-carbon compound called 3-phosphoglycerate, or 3-PGA.
3-PGA is a more stable molecule, and represents the initial "fixed" carbon that the plant can now use for sugar synthesis. These 3-PGA molecules then enter the reduction phase of the Calvin Cycle, marking the next crucial step in creating the energy-rich sugars that power the plant, and ultimately, the world.
The Reduction Phase: Converting 3-PGA to G3P
Following the crucial carbon fixation step, the Calvin Cycle progresses into the reduction phase, a stage where the newly acquired carbon is transformed into a usable sugar. This transformation demands a significant energy input, supplied by the products of the light-dependent reactions, to drive the conversion of 3-PGA into G3P.
Harnessing Energy from ATP
The light-dependent reactions, occurring in the thylakoid membranes, are pivotal in capturing solar energy and converting it into chemical energy. This chemical energy is primarily stored in the form of ATP (Adenosine Triphosphate) and NADPH. ATP serves as the immediate energy currency of the cell.
In the context of the Calvin Cycle, ATP's role is to energize the 3-PGA molecules. This is achieved through phosphorylation, where a phosphate group from ATP is transferred to 3-PGA, creating an activated intermediate. This phosphorylation is essential for the next step, where NADPH comes into play.
Leveraging Reducing Power from NADPH
NADPH (Nicotinamide Adenine Dinucleotide Phosphate), is the other key energy-carrying molecule generated during the light-dependent reactions. It acts as a powerful reducing agent, meaning it donates electrons to other molecules.
During the reduction phase, NADPH donates its electrons to the phosphorylated 3-PGA intermediate. This electron transfer reduces the intermediate, ultimately converting it into G3P (Glyceraldehyde-3-phosphate). This reaction is vital because it represents the initial point where inorganic carbon is converted into an organic sugar.
The Significance of G3P
G3P stands as a pivotal molecule within the Calvin Cycle and, indeed, within plant metabolism as a whole. As a three-carbon sugar, it serves as the primary product of the Calvin Cycle and the starting point for the synthesis of more complex carbohydrates.
Some G3P molecules are diverted to regenerate RuBP, ensuring the continuation of the Calvin Cycle. Others are used to synthesize a variety of sugars, including glucose, fructose, sucrose, and starch. These sugars serve as the building blocks for plant structures and as a source of energy for cellular processes.
The production of G3P during the reduction phase marks a critical juncture in photosynthesis. It represents the culmination of energy input from the light-dependent reactions and the successful conversion of inorganic carbon into a biologically useful form. This accomplishment sets the stage for the final phase of the Calvin Cycle, where the initial CO2 acceptor, RuBP, is regenerated.
Regeneration: Recreating RuBP to Sustain the Cycle
Following the reduction of 3-PGA to G3P, the Calvin Cycle faces a critical juncture: regenerating RuBP. This seemingly less glamorous phase is, in fact, absolutely vital for sustained carbon fixation and, consequently, for the entire photosynthetic process. Without RuBP regeneration, the cycle grinds to a halt, effectively shutting down carbon assimilation.
The Indispensable Role of RuBP Regeneration
RuBP (Ribulose-1,5-bisphosphate) acts as the initial acceptor of carbon dioxide. It's the molecule that kickstarts the entire Calvin Cycle.
Therefore, its continuous availability is paramount. Regeneration ensures that the cycle doesn't deplete its RuBP reserves, allowing for the continuous fixation of atmospheric CO2. This, in turn, allows for the continuous production of sugars.
Essentially, RuBP regeneration is the engine that keeps the Calvin Cycle running, transforming it from a one-time event into a self-sustaining, cyclical process.
A Complex Web of Reactions
The regeneration of RuBP from G3P is not a simple, single-step conversion. It's a complex and intricate series of enzymatic reactions.
These reactions involve a diverse array of sugar molecules, including three-carbon, four-carbon, five-carbon, six-carbon, and seven-carbon sugars. These are meticulously shuffled and rearranged.
This process requires the coordinated action of multiple enzymes, each catalyzing a specific reaction in the pathway. The complexity of this network highlights the sophisticated biochemical machinery at play within the chloroplast.
The process involves reactions like transketolase and transaldolase reactions. These meticulously rearrange carbon atoms between sugar molecules.
Ultimately, through a carefully orchestrated series of steps, five molecules of G3P are converted into three molecules of RuBP, effectively replenishing the cycle's starting material.
The Energy Investment in Regeneration
The regeneration phase is not only complex in terms of biochemical transformations but also demands a significant energy input. This energy is supplied by ATP, which, as we know, is generated during the light-dependent reactions of photosynthesis.
Specifically, ATP is used to phosphorylate ribulose-5-phosphate, converting it into RuBP. This phosphorylation step is essential for regenerating the CO2 acceptor molecule.
Without sufficient ATP, the regeneration process slows down, limiting the overall rate of carbon fixation. This underscores the crucial link between the light-dependent reactions, which generate ATP, and the Calvin Cycle, which utilizes that ATP to drive carbon assimilation.
[Regeneration: Recreating RuBP to Sustain the Cycle Following the reduction of 3-PGA to G3P, the Calvin Cycle faces a critical juncture: regenerating RuBP. This seemingly less glamorous phase is, in fact, absolutely vital for sustained carbon fixation and, consequently, for the entire photosynthetic process. Without RuBP regeneration, the cycle grinds to a halt. But G3P's importance doesn't end there, as it also has another significant purpose to fill.]
Products of the Calvin Cycle: From G3P to Complex Sugars
G3P, or glyceraldehyde-3-phosphate, stands as the primary direct product of the Calvin Cycle. However, its role extends far beyond being a mere endpoint. This three-carbon sugar molecule serves as a crucial metabolic intermediate, a branching point from which the plant orchestrates a diverse array of biochemical pathways. Some G3P molecules are reinvested within the cycle to regenerate RuBP, ensuring the perpetuation of carbon fixation. Others are channeled towards the synthesis of more complex carbohydrates, fueling plant growth and providing energy reserves. Understanding the dual fates of G3P is key to appreciating the elegance and efficiency of the Calvin Cycle.
G3P's Two Fates: Regeneration vs. Sugar Synthesis
The fate of each G3P molecule produced in the Calvin Cycle is essentially a carefully balanced decision. The plant must allocate enough G3P to RuBP regeneration to maintain the cycle's operation and carbon fixation capacity.
However, any G3P produced in excess of that requirement is available for conversion into more complex carbohydrates. This precise balancing act allows the plant to adapt to fluctuating environmental conditions and changing metabolic demands.
If the plant needs more energy, G3P will be primarily directed toward sugar synthesis. If the plant is low in carbon, then it will use the G3P to regenerate RuBP.
From G3P to Complex Carbohydrates: Building Blocks of Life
The real magic of the Calvin Cycle lies not just in fixing carbon, but in the potential of its product, G3P, to be transformed into a multitude of other essential compounds.
G3P serves as the foundation for the synthesis of glucose and fructose, two simple sugars that are often considered the primary energy currencies of the plant.
These monosaccharides can then be combined to form disaccharides like sucrose, the form in which sugar is often transported throughout the plant's vascular system.
Glucose: The Foundation of Energy Storage
Glucose is often considered to be the most important sugar for plants. It serves as the direct precursor for cellulose, the main structural component of plant cell walls. Cellulose provides rigidity and support to the plant.
Fructose: The Sweet Partner to Glucose
Fructose, another monosaccharide produced from G3P, partners with glucose to form sucrose. It is also used in other processes, especially when the plant is making fruit. The fructose helps to produce a sweet and enjoyable fruit, which encourages animals to disperse its seeds.
Sucrose: Transporting Energy Throughout the Plant
Sucrose is a disaccharide formed by combining glucose and fructose. It is readily transported throughout the plant via the phloem, providing energy to tissues and organs that are not actively photosynthesizing.
Starch: Long-Term Energy Storage
When energy is abundant, plants convert excess glucose into starch, a complex polysaccharide composed of long chains of glucose molecules. Starch is a highly efficient form of energy storage, allowing plants to accumulate reserves that can be mobilized when needed, such as during periods of darkness or stress. Starch is the predominant form of carbohydrate storage in many plant tissues, including roots, tubers, and seeds.
Ultimately, the Calvin Cycle, through the pivotal role of G3P, provides the fundamental building blocks for plant growth, development, and survival. It is a testament to the intricate and interconnected nature of biochemical processes within living organisms.
The Calvin Cycle's Integration with Photosynthesis: A Complete Picture
Regeneration: Recreating RuBP to Sustain the Cycle Following the reduction of 3-PGA to G3P, the Calvin Cycle faces a critical juncture: regenerating RuBP. This seemingly less glamorous phase is, in fact, absolutely vital for sustained carbon fixation and, consequently, for the entire photosynthetic process. Without RuBP regeneration, the cycle grinds to a halt, effectively stopping sugar production.
The Interdependent Dance of Light and Dark
The Calvin Cycle, often referred to as the "dark reactions" or "light-independent reactions," does not operate in isolation. It is inextricably linked to the light-dependent reactions of photosynthesis. This connection is critical.
The light-dependent reactions, occurring in the thylakoid membranes, capture light energy and convert it into chemical energy in the form of ATP and NADPH. These two molecules are the sole energy source fueling the Calvin Cycle.
ATP provides the necessary phosphate groups for various phosphorylation reactions, while NADPH acts as a reducing agent, donating electrons to convert 3-PGA into G3P. Therefore, without the constant supply of ATP and NADPH from the light-dependent reactions, the Calvin Cycle cannot proceed.
The light-dependent reactions are the power source, and the Calvin Cycle is the engine, working together to convert light energy into stable chemical energy.
Photosynthesis: From Sunlight to Sugars
Photosynthesis, in its entirety, is a remarkable process by which plants and other organisms convert light energy into chemical energy. It can be summarized as a two-stage process.
First, the light-dependent reactions capture solar energy and convert it into the chemical energy of ATP and NADPH. Oxygen is released as a byproduct during this stage.
Second, the Calvin Cycle utilizes the ATP and NADPH produced in the first stage to fix atmospheric CO2 and synthesize sugars, primarily G3P. These sugars serve as the foundation for building more complex carbohydrates, such as glucose, sucrose, and starch.
Thus, photosynthesis is a carefully orchestrated sequence of events that captures light energy, converts it into chemical energy, and stores it in the form of sugars. These sugars then fuel plant growth, development, and overall metabolism.
C3 Photosynthesis: A Foundation for Life
The Calvin Cycle is the central component of what is known as C3 photosynthesis. This is the most common photosynthetic pathway used by plants, responsible for the vast majority of carbon fixation on Earth.
In C3 photosynthesis, the initial carbon fixation step involves the enzyme RuBisCO catalyzing the reaction between CO2 and RuBP to form a three-carbon compound (3-PGA). It is from this three-carbon compound that the term "C3 photosynthesis" originates.
However, C3 photosynthesis is not without its limitations. RuBisCO, under certain conditions (high oxygen concentrations and high temperatures), can also bind to oxygen instead of CO2, leading to a process called photorespiration. This reduces the efficiency of carbon fixation.
Despite this limitation, C3 photosynthesis remains the primary mechanism by which plants incorporate atmospheric carbon into the biosphere, underpinning the food chain and supporting life on Earth. It stands as a fundamental biochemical pathway, driving global carbon cycling and playing a pivotal role in maintaining Earth's ecosystems.
Challenges to the Calvin Cycle: Understanding Photorespiration
Following the intricate steps of carbon fixation, reduction, and RuBP regeneration, the Calvin Cycle encounters a significant hurdle: photorespiration. This process, seemingly counterproductive, can dramatically reduce the efficiency of carbon fixation, especially under certain environmental conditions. Understanding photorespiration is crucial for comprehending the limitations of C3 photosynthesis and the evolutionary adaptations that have arisen to mitigate its effects.
What is Photorespiration?
Photorespiration, also known as C2 photosynthesis, is a metabolic pathway that occurs in plants when the enzyme RuBisCO (ribulose-1,5-bisphosphate carboxylase/oxygenase) oxygenates RuBP (ribulose-1,5-bisphosphate) instead of carboxylating it. This seemingly subtle change has profound consequences.
Instead of producing two molecules of 3-PGA (3-phosphoglycerate), as it does in the Calvin Cycle, RuBisCO produces one molecule of 3-PGA and one molecule of phosphoglycolate.
Phosphoglycolate is then processed through a series of reactions in the chloroplast, peroxisome, and mitochondrion, ultimately releasing CO2.
This process consumes ATP and NADPH without producing any sugars, effectively undoing some of the work of photosynthesis.
In essence, photorespiration wastes energy and carbon, diminishing the net photosynthetic output of the plant.
The Conditions Favoring Photorespiration
Several environmental factors can influence the rate of photorespiration relative to carbon fixation. The most significant is the ratio of CO2 to O2 in the leaf.
High oxygen concentrations and low carbon dioxide concentrations favor the oxygenation reaction catalyzed by RuBisCO, leading to increased photorespiration.
These conditions often arise in hot, dry environments, where plants close their stomata to conserve water.
Closing the stomata restricts CO2 entry into the leaf while allowing O2, produced during the light-dependent reactions, to build up.
High temperatures also increase RuBisCO's affinity for O2, further exacerbating the problem.
RuBisCO's Dual Nature: Carboxylase vs. Oxygenase
The root of photorespiration lies in the active site of RuBisCO, the enzyme responsible for both carbon fixation and oxygenation of RuBP.
RuBisCO, despite its central role in photosynthesis, is not a perfect enzyme.
Its active site can bind to both CO2 and O2, and the binding affinity is influenced by their relative concentrations.
When CO2 levels are high, RuBisCO efficiently carboxylates RuBP, initiating the Calvin Cycle.
However, when O2 levels are high, RuBisCO can mistakenly bind O2 to RuBP, initiating the photorespiratory pathway.
This dual nature of RuBisCO is a critical point in understanding why photorespiration occurs and why it presents a challenge to photosynthetic efficiency.
The evolutionary history of RuBisCO, predating the rise of atmospheric oxygen, helps explain its imperfect specificity.
In the early Earth's atmosphere, CO2 concentrations were much higher, and O2 concentrations were much lower, so there was little selective pressure for RuBisCO to discriminate strongly against O2.
Implications for Plant Productivity
Photorespiration can significantly reduce plant productivity, especially in hot, arid climates.
In some plants, photorespiration can decrease photosynthetic efficiency by as much as 50%.
This reduction in efficiency can have significant consequences for plant growth, development, and yield.
Plants have evolved various mechanisms to minimize the negative impacts of photorespiration, including C4 and CAM photosynthesis, which concentrate CO2 around RuBisCO, effectively outcompeting O2.
Understanding photorespiration is essential for developing strategies to improve crop yields and enhance plant resilience to environmental stresses.
Video: Calvin Cycle Outputs: What Plants Produce
Frequently Asked Questions: Calvin Cycle Outputs
What are the main products generated by the Calvin cycle?
The primary calvin cycle outputs are glyceraldehyde-3-phosphate (G3P), a three-carbon sugar. G3P is used to make glucose, fructose, and other essential organic molecules. These sugars provide energy and building blocks for the plant's growth and metabolism.
Besides sugars, does the Calvin cycle produce anything else essential?
Yes. A crucial, often overlooked, calvin cycle output is the regeneration of ribulose-1,5-bisphosphate (RuBP). RuBP is the initial carbon dioxide acceptor, allowing the cycle to continue fixing CO2. Without RuBP regeneration, the cycle would quickly halt.
What happens to the sugars created by the Calvin cycle outputs?
The glyceraldehyde-3-phosphate (G3P) produced as calvin cycle outputs is primarily used for two purposes. A portion is used to regenerate RuBP to keep the cycle running. The remaining G3P is converted into other carbohydrates like glucose and starch.
How do the Calvin cycle outputs contribute to a plant's overall survival?
The calvin cycle outputs, specifically the sugars, provide the plant with the energy and carbon skeletons it needs to build its cell walls, synthesize proteins, and perform all other life processes. These sugars are the foundation for plant biomass and growth, directly impacting survival.
So, there you have it! Now you know the secret behind the sugar factories humming inside every plant. From carbon dioxide and a little bit of energy, they whip up the magic of G3P, later used for glucose and other goodies! Remember, these Calvin cycle outputs are the building blocks for pretty much everything else a plant needs to grow and thrive, and ultimately, what sustains a whole lot of life on Earth. Pretty cool, huh?