Boron Valence Electrons: Chemistry Role & Compounds
Boron, a metalloid element, exhibits unique chemical behaviors attributable to its electronic configuration, particularly concerning its boron valence electrons. Linus Pauling's work on chemical bonding significantly contributed to our understanding of how these valence electrons influence boron's ability to form covalent compounds. These compounds, often synthesized and analyzed in laboratory settings, such as those equipped with spectroscopic tools from companies like Agilent Technologies, play a crucial role in various industrial applications. The electronic structure of boron and the resulting behavior of boron valence electrons dictates its chemistry, leading to the formation of electron-deficient compounds and clusters.
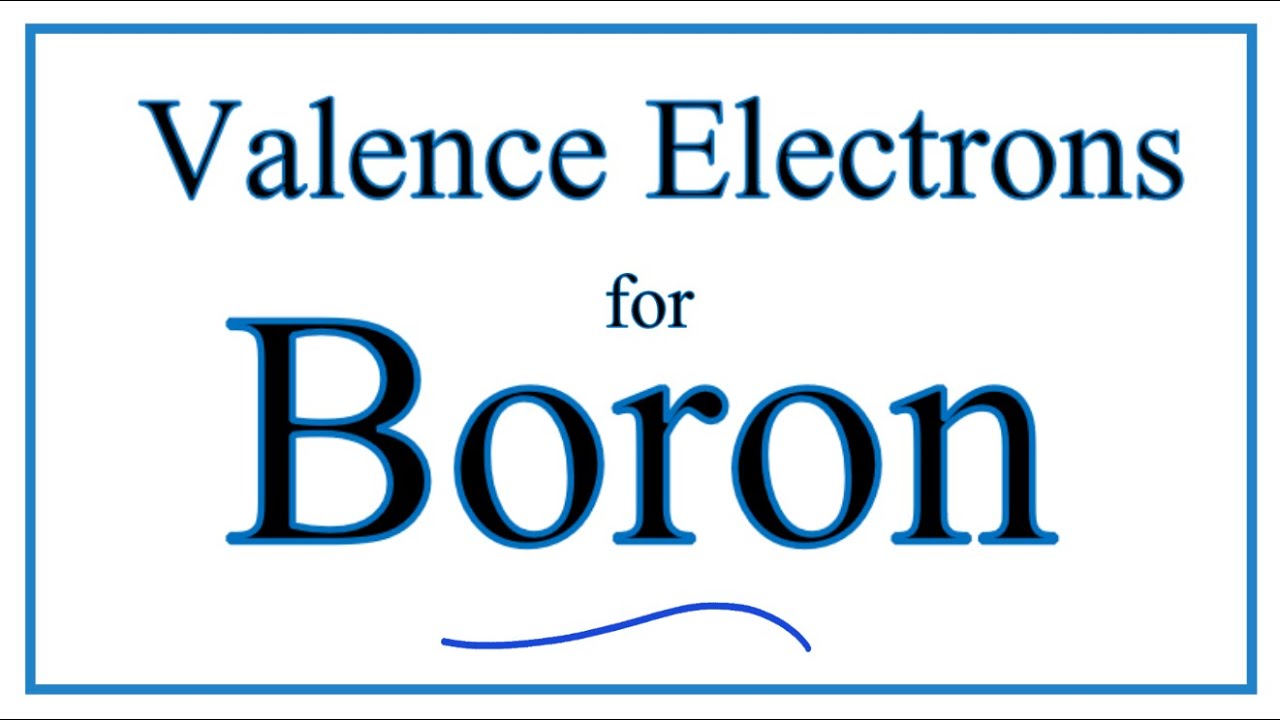
Image taken from the YouTube channel Wayne Breslyn (Dr. B.) , from the video titled How to Find the Valence Electrons for Boron (B) .
Boron, a metalloid element nestled in Group 13 of the periodic table, often evades the spotlight. However, a closer examination reveals a chemical landscape of remarkable versatility and unparalleled significance.
Boron's unique electronic configuration and diminutive atomic size underpin a plethora of unconventional bonding motifs and structural arrangements. These features are not mere curiosities but rather the foundation for boron's pervasive influence across diverse scientific disciplines.
The Broad Impact of Boron Chemistry
The implications of understanding boron chemistry are far-reaching. In materials science, boron is indispensable in the creation of high-performance ceramics, lightweight alloys, and novel semiconductors. Its capacity to form robust networks and modulate electronic properties makes it a critical ingredient in advanced materials.
Organic synthesis, too, has been revolutionized by the advent of boron-containing reagents. Reactions like the Suzuki-Miyaura coupling, which relies on boron-containing organic compounds, exemplify its power in constructing complex molecules with exquisite control.
Navigating the Boron Landscape: A Roadmap
This exploration delves into the core principles that govern boron's chemical behavior. We will navigate from the foundational properties of the element to the intricacies of borane clusters and the diverse applications of boron-containing compounds.
Prepare to journey through the seemingly strange, yet incredibly useful, world of boron chemistry.
Fundamental Properties of Boron: A Building Block of Reactivity
Boron, a metalloid element nestled in Group 13 of the periodic table, often evades the spotlight. However, a closer examination reveals a chemical landscape of remarkable versatility and unparalleled significance.
Boron's unique electronic configuration and diminutive atomic size underpin a plethora of unconventional bonding motifs and structural arrangements. These, in turn, dictate its reactivity and propensity to form a diverse array of compounds. Let's delve into the fundamental properties that govern boron's chemical behavior.
Electronic Structure and Bonding
Boron possesses a ground-state electron configuration of 1s²2s²2p¹. This configuration reveals the presence of three valence electrons, which are crucial for its bonding interactions.
The relatively high effective nuclear charge experienced by these valence electrons contributes to boron's characteristic behavior.
Valence Electrons: The Key to Boron's Reactivity
Boron's three valence electrons are the actors in its chemical performances. They determine the number and types of bonds boron can form with other elements.
However, it's equally important to recognize what it lacks – a full octet. This deficiency drives boron's unique reactivity.
Electron Configuration: A Detailed Look
A deeper dive into boron's electron configuration necessitates examining the distribution of electrons within its atomic orbitals.
The 1s orbital, closest to the nucleus, is fully occupied with two electrons. The 2s orbital also houses two electrons, while the 2p orbital contains a single electron.
This configuration, often represented as [He]2s²2p¹, dictates the spatial arrangement and energy levels of boron's valence electrons.
Electronegativity and Ionization Energy
Boron's electronegativity and ionization energy also significantly influence its bonding characteristics.
Electronegativity: The Tug-of-War
Boron exhibits a moderate electronegativity, positioned between electropositive metals and highly electronegative nonmetals.
This intermediate electronegativity dictates the types of bonds boron tends to form. It engages in covalent bonding with elements of similar electronegativity and polar covalent bonding with more electronegative elements.
Ionization Energy: Reluctance to Lose
The ionization energy of boron, while not exceptionally high, reflects the energy required to remove an electron from the atom.
This value influences boron's tendency to form positive ions. Boron is less likely to form stable B³⁺ ions under normal chemical conditions due to the energy cost associated with removing three electrons.
Oxidation State and Electron Deficiency
These two concepts are deeply intertwined and central to understanding boron's chemistry.
Oxidation State: Embracing +3
Boron predominantly exhibits a +3 oxidation state in its compounds. This stems from its tendency to share or donate its three valence electrons to achieve a more stable electronic configuration.
The +3 oxidation state is observed in a wide range of boron compounds, including boron oxides, halides, and organoboron compounds.
Electron Deficiency: The Driving Force
Perhaps the most defining characteristic of boron is its electron deficiency. Having only three valence electrons, boron struggles to achieve a complete octet in its bonding arrangements.
This inherent electron deficiency has profound implications for the structure and reactivity of boron compounds.
Octet Rule and Hybridization
Boron's defiance of the octet rule is directly linked to its hybridization patterns.
Octet Rule: A Notable Exception
Unlike many elements that strive to achieve a full octet of electrons in their valence shell, boron frequently breaks the octet rule.
This deviation from the norm is a direct consequence of its electron deficiency, leading to unusual bonding arrangements and reactivity patterns.
Hybridization: Shaping Molecular Geometry
Boron's hybridization plays a pivotal role in determining the geometry of its compounds. Boron commonly adopts either sp² or sp³ hybridization.
- sp² hybridization: Results in a trigonal planar geometry with bond angles of approximately 120 degrees. This is observed in compounds like BF₃.
- sp³ hybridization: Leads to a tetrahedral geometry with bond angles of approximately 109.5 degrees. This is present when boron forms four single bonds.
Lewis Acidity: The Electron Acceptor
Boron compounds are quintessential Lewis acids due to their electron deficiency.
Lewis Acids: Seeking Electrons
Boron compounds act as Lewis acids, readily accepting electron pairs from Lewis bases to form adducts.
This electron-accepting behavior is pivotal in numerous chemical reactions and catalytic processes.
The Lewis acidity of boron compounds is directly related to the electron deficiency of the boron atom. It makes them valuable catalysts and reagents in organic synthesis and other fields.
Boron Hydrides (Boranes): Exploring Unusual Bonding
Having established the fundamental properties that govern boron's reactivity, we now turn our attention to a class of compounds that truly showcase the element's unconventional nature: boron hydrides, more commonly known as boranes. These compounds, formed from boron and hydrogen, defy traditional bonding models and present a fascinating challenge to our understanding of chemical structure and reactivity.
A Glimpse into the Borane Landscape
Boranes are characterized by their electron-deficient nature, which leads to the formation of unusual bonding arrangements not typically observed in other areas of chemistry. Unlike hydrocarbons where each carbon atom typically forms four bonds, boron atoms in boranes often form fewer than four bonds, leading to a deficiency of electrons and the formation of unique structures.
Let's examine some representative examples to appreciate the structural diversity and bonding peculiarities of boranes:
- Diborane (B2H6): Perhaps the most well-known borane, diborane features two bridging hydrogen atoms between the two boron atoms. This structure deviates significantly from the expectation of simple covalent bonds.
- Pentaborane (B5H9): This borane exhibits a more complex cage-like structure, with five boron atoms arranged in a square pyramidal geometry. This showcases the ability of boron to form multi-center bonds.
- Decaborane (B10H14): Decaborane presents an even more intricate structure, a fragment of an icosahedron. It consists of ten boron atoms and fourteen hydrogen atoms, further exemplifying the capacity of boron to generate complex polyhedral structures.
The Enigmatic Three-Center Two-Electron Bond
The key to understanding the unusual structures and bonding in boranes lies in the concept of three-center two-electron (3c-2e) bonds. This type of bond, uncommon in traditional organic chemistry, is critical for stabilizing electron-deficient boron compounds.
Formation and Stability of 3c-2e Bonds
In a typical covalent bond, two atoms share two electrons. However, in a 3c-2e bond, three atoms share only two electrons. This occurs when a hydrogen atom bridges two boron atoms, forming a B-H-B bridge.
The two electrons are delocalized over the three atoms (two boron, one hydrogen), creating a bonding interaction that stabilizes the structure despite the electron deficiency. The stability arises from the simultaneous interaction of all three atoms, effectively distributing the electron density and lowering the overall energy of the molecule. This type of bonding is crucial for the existence of boranes, as it allows boron to achieve a degree of electronic satisfaction despite its inherent electron deficiency.
Visualizing the 3c-2e Bond
Imagine a hydrogen atom positioned between two boron atoms, equidistant from each. The hydrogen's 1s orbital overlaps with the sp3 hybrid orbitals of the two boron atoms. This overlap forms a molecular orbital encompassing all three atoms. The two electrons reside in this molecular orbital, effectively bonding all three atoms together. Diagrams illustrating this electron delocalization further clarify the essence of the 3c-2e bond.
Boron-Containing Compounds: A Diverse Family
Having explored the intriguing world of boranes and their unconventional bonding, we now expand our scope to encompass the broader landscape of boron-containing compounds. These substances exhibit a remarkable diversity in structure, properties, and applications, underscoring the pivotal role of boron in various chemical disciplines. From industrial materials to essential reagents in organic synthesis, boron's versatility is truly remarkable.
This section will delve into several key representatives of this diverse family, highlighting their unique characteristics and the significance of their contributions to science and technology.
Boron Oxides: The Foundation of Borosilicate Glass
Boron trioxide (B2O3) stands as a fundamental boron oxide, characterized by its glassy nature and its crucial role in the creation of borosilicate glass, such as Pyrex.
The structure of B2O3 features a network of interconnected BO3 triangles. The presence of boron in the glass matrix imparts enhanced thermal shock resistance and chemical durability. This is invaluable for laboratory glassware and cookware.
Applications in Glass Manufacturing
The addition of boron trioxide to glass formulations significantly reduces the coefficient of thermal expansion. This makes borosilicate glass less prone to cracking under rapid temperature changes. This property is critical in applications demanding high thermal stability.
Boric Acid: Antiseptic and More
Boric acid (H3BO3), a weak Lewis acid, manifests as a white, crystalline solid with mild antiseptic, insecticidal, and flame retardant properties.
Its acidity stems from its ability to accept hydroxide ions (OH-) from water, generating tetrahydroxyborate anions [B(OH)4]-.
Medical and Insecticidal Applications
In medicine, boric acid finds use as an antiseptic for minor burns and cuts.
Its insecticidal properties render it effective in controlling cockroaches, ants, and other pests. Boric acid disrupts their metabolism and desiccates their exoskeletons.
Boron Nitride: A Multifaceted Ceramic Material
Boron nitride (BN) exists in several allotropic forms, each exhibiting distinct properties and applications.
The two most prominent allotropes are hexagonal boron nitride (h-BN), and cubic boron nitride (c-BN).
Hexagonal Boron Nitride (h-BN): The White Graphite
Analogous in structure to graphite, h-BN consists of stacked layers of fused B-N hexagons.
It boasts exceptional thermal and chemical stability, rendering it an excellent lubricant and high-temperature insulator.
Cubic Boron Nitride (c-BN): The Hardest Material After Diamond
Cubic boron nitride, with its diamond-like structure, ranks as the second hardest material known, after diamond.
Its extreme hardness and thermal conductivity make it ideal for abrasive and cutting tools. It excels in machining hardened steel and other difficult-to-cut materials.
Boron Halides: Potent Lewis Acids in Catalysis
Boron halides, such as boron trifluoride (BF3) and boron trichloride (BCl3), exemplify strong Lewis acids due to boron's electron deficiency.
Their potent electron-accepting ability makes them versatile catalysts in a wide array of chemical reactions.
Catalytic Applications
Boron halides facilitate Friedel-Crafts alkylations and acylations, polymerizations, and various other organic transformations.
Their catalytic activity stems from their capacity to activate reactants through coordination and stabilization of transition states.
Organoboron Compounds: Cornerstones of Organic Synthesis
Organoboron compounds, featuring direct carbon-boron bonds, have revolutionized organic synthesis.
Their utility lies in their ability to participate in a diverse range of reactions with high selectivity and efficiency.
Suzuki-Miyaura Coupling
The Suzuki-Miyaura coupling stands as a hallmark reaction employing organoboron compounds. It involves the palladium-catalyzed cross-coupling of an organoboron compound with an organic halide or pseudohalide.
This reaction enables the formation of carbon-carbon bonds. It is extensively utilized in the synthesis of pharmaceuticals, agrochemicals, and materials.
Borazines: Inorganic Analogues of Benzene
Borazines, with the formula (B3N3H6), represent inorganic analogues of benzene.
The borazine ring consists of alternating boron and nitrogen atoms, mimicking the hexagonal structure of benzene.
Structural and Electronic Properties
While borazines share structural similarities with benzene, their electronic properties differ due to the electronegativity difference between boron and nitrogen. The B-N bonds are polarized, leading to a partial positive charge on boron and a partial negative charge on nitrogen.
This polarity influences the reactivity of borazines compared to benzene. It renders them susceptible to nucleophilic attack at boron and electrophilic attack at nitrogen.
Applications and Significance: Why Boron Chemistry Matters
Having explored the intriguing world of boranes and their unconventional bonding, we now expand our scope to encompass the broader landscape of boron-containing compounds. These substances exhibit a remarkable diversity in structure, properties, and applications, underscoring the pivotal role of boron in numerous technological and scientific advancements. Let us delve into the key applications of boron chemistry across various industries and consider the exciting prospects for future research.
Boron compounds are not merely laboratory curiosities; they are integral components in a wide array of practical applications. From enhancing material properties to enabling sophisticated chemical transformations, the versatility of boron chemistry is undeniable.
Industrial Applications of Boron Compounds
Boron compounds are key components in numerous industrial sectors. Borax (sodium borate) is a cornerstone of the detergent industry, where it enhances cleaning power and stabilizes enzymatic formulations.
Boron fibers, renowned for their high tensile strength and lightweight nature, are critical in aerospace engineering. They find application in reinforcing composite materials used in aircraft structures and other high-performance components.
Enhancing Material Properties
In metallurgy, boron serves as a crucial alloying agent. Small additions of boron can significantly improve the hardenability and wear resistance of steel. This enhancement is achieved through the formation of hard boride phases within the steel matrix, which inhibit grain growth and increase overall strength.
Boron carbide (B4C), one of the hardest materials known, is widely used as an abrasive in grinding and polishing applications. Its exceptional hardness also makes it ideal for neutron absorption in nuclear reactors, serving as a critical safety component.
Boron in Agriculture
Boron plays a pivotal, albeit often underestimated, role in plant nutrition. As a micronutrient, it is essential for cell wall development, carbohydrate metabolism, and reproductive processes in plants.
Boron deficiency in soils can lead to stunted growth and reduced crop yields. Therefore, boron-containing fertilizers are routinely used to ensure optimal plant health and productivity in various agricultural settings.
Ongoing Research and Future Directions
The field of boron chemistry is far from static; it remains a vibrant area of ongoing research and innovation. Current investigations are pushing the boundaries of what is possible with boron-containing materials and exploring their potential in emerging technologies.
Boron Neutron Capture Therapy (BNCT)
Boron neutron capture therapy (BNCT) is a promising cancer treatment modality. It relies on the ability of boron-10 to selectively accumulate in tumor cells. Subsequent irradiation with low-energy neutrons causes boron-10 to undergo a nuclear reaction, releasing highly energetic alpha particles that selectively destroy cancerous cells while sparing healthy tissue.
Boron-Based Polymers and Materials
Researchers are actively exploring the synthesis and characterization of novel boron-based polymers. These materials exhibit unique properties such as self-healing capabilities, stimuli-responsiveness, and high-temperature stability.
Such polymers hold promise for applications in advanced adhesives, coatings, and structural materials.
Catalysis and Organic Synthesis
Organoboron compounds continue to be indispensable tools in organic synthesis. Their unique reactivity enables the construction of complex molecules with high precision and efficiency.
Ongoing research focuses on developing new organoboron catalysts for a wide range of chemical transformations, including C-C bond formation, oxidation reactions, and asymmetric synthesis.
The Importance of Continued Exploration
The continued exploration of boron chemistry is of paramount importance for several reasons. Boron's unique electronic structure and bonding characteristics provide a rich playground for discovering novel materials and chemical reactions.
Investing in boron research will undoubtedly lead to groundbreaking discoveries and technological advancements that will benefit society in profound ways. The potential rewards are simply too significant to ignore.
From advanced materials to life-saving therapies, boron chemistry holds the key to solving some of the world's most pressing challenges. Its continued exploration is not just a scientific endeavor; it is an investment in a brighter future.
Video: Boron Valence Electrons: Chemistry Role & Compounds
FAQs: Boron Valence Electrons, Chemistry & Compounds
Why does boron often form electron-deficient compounds?
Boron has only three boron valence electrons. This means it typically forms only three covalent bonds. Satisfying the octet rule would require eight electrons, so boron often exists in compounds where it doesn't have a full octet, leading to electron deficiency and a tendency to accept electron pairs.
How do boron valence electrons contribute to its bonding versatility?
While boron is electron-deficient, its three boron valence electrons allow it to form a variety of bonds. It can form traditional covalent bonds, but also participates in coordinate covalent bonds by accepting electron pairs from other atoms or molecules, expanding its bonding possibilities.
What impact do boron valence electrons have on the properties of boranes?
Boranes are compounds of boron and hydrogen, known for their unusual structures and bonding. The limited number of boron valence electrons leads to these unique bonding arrangements, involving multi-center bonds where electrons are delocalized across multiple atoms. This is quite different from typical two-center, two-electron bonds.
What types of compounds does boron form, and what role do boron valence electrons play?
Boron forms compounds like borax (used in cleaning), boric acid (antiseptic), and boron nitride (abrasive). In these compounds, boron valence electrons participate in forming strong covalent bonds with oxygen, nitrogen, and other elements, dictating the compound's stability and its physical/chemical characteristics.
So, there you have it! Boron valence electrons might seem like a niche topic, but they're actually crucial for understanding how boron behaves and forms so many interesting compounds. Hopefully, this has given you a solid foundation for diving deeper into the fascinating world of boron chemistry!