Boiling Point: What Factors Cause Variations?
The boiling point of a substance, a critical parameter in chemistry and chemical engineering, is not a fixed value, as it is susceptible to variations induced by several factors. Intermolecular forces, specifically the strength of attractions between molecules, dictate the energy required for a substance to transition from liquid to gas. Pressure, an extrinsic condition imposed on the liquid, plays a vital role, where decreased atmospheric pressure lowers the boiling point, a principle leveraged in processes such as vacuum distillation within industrial settings. Therefore, understanding what factors can cause a varied boiling point is essential for predicting the behavior of substances under diverse conditions and is a fundamental aspect in the design and optimization of chemical processes.
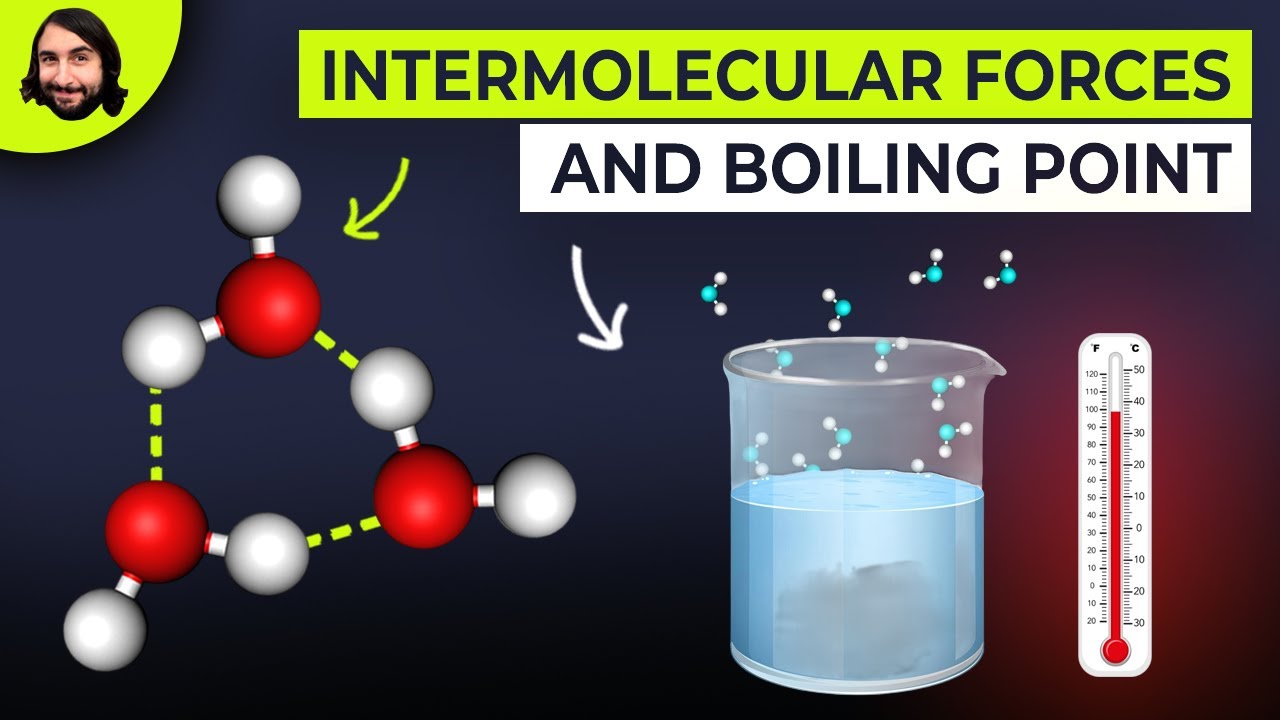
Image taken from the YouTube channel Professor Dave Explains , from the video titled Intermolecular Forces and Boiling Points .
The boiling point of a substance represents a critical physical property, dictating the temperature at which it transitions from a liquid to a gaseous state. This phenomenon is not merely a point on a thermometer; it's a window into the intricate world of molecular interactions and energy dynamics. Understanding boiling points is essential across various scientific disciplines, from chemical synthesis to material science, and even plays a crucial role in everyday processes like cooking.
Defining Boiling Point Elevation
Boiling point elevation is a colligative property, meaning it depends on the concentration of solute particles in a solution, rather than the identity of those particles. When a non-volatile solute is added to a solvent, the boiling point of the resulting solution is higher than that of the pure solvent.
This elevation occurs because the solute particles effectively dilute the solvent, reducing its vapor pressure. Consequently, a higher temperature is required to achieve a vapor pressure equal to the surrounding atmospheric pressure, thereby raising the boiling point. This principle finds practical application in antifreeze solutions, where solutes prevent water from freezing or boiling over in extreme temperatures.
The Fundamental Relationship to Vapor Pressure
Boiling occurs when the vapor pressure of a liquid equals the external pressure exerted on it. Vapor pressure is the pressure exerted by a vapor in thermodynamic equilibrium with its condensed phases (solid or liquid) at a given temperature in a closed system.
A liquid's vapor pressure increases with temperature. When this vapor pressure matches the surrounding atmospheric pressure, bubbles of vapor form within the liquid and rise to the surface—the process we know as boiling.
The boiling point is therefore pressure-dependent; at higher altitudes, where atmospheric pressure is lower, liquids boil at lower temperatures. Conversely, under increased pressure, the boiling point rises.
The Role of Intermolecular Forces (IMFs)
Intermolecular forces (IMFs) are the attractive or repulsive forces that exist between molecules. These forces play a critical role in determining the physical properties of substances, including their boiling points. Stronger IMFs require more energy to overcome, leading to higher boiling points.
There are several types of IMFs, including:
-
London Dispersion Forces (LDFs): Present in all molecules, these are temporary, weak attractions arising from instantaneous fluctuations in electron distribution. They are more significant in larger molecules with more electrons.
-
Dipole-Dipole Interactions: Occur between polar molecules that have a permanent dipole moment due to uneven electron sharing.
-
Hydrogen Bonding: A particularly strong type of dipole-dipole interaction that occurs when hydrogen is bonded to highly electronegative atoms like oxygen, nitrogen, or fluorine. Hydrogen bonds significantly elevate boiling points.
The strength and type of IMFs present in a substance directly dictate the amount of energy (heat) needed to transition it from the liquid to the gaseous phase, thus influencing its boiling point.
Decoding the Influences: Factors That Determine Boiling Point
The boiling point of a substance represents a critical physical property, dictating the temperature at which it transitions from a liquid to a gaseous state. This phenomenon is not merely a point on a thermometer; it's a window into the intricate world of molecular interactions and energy dynamics. Understanding boiling points is essential across various scientific disciplines, from chemical synthesis to material science. Several key factors influence a substance's boiling point, each playing a distinct role in determining the thermal energy required for phase transition.
The Potent Influence of Hydrogen Bonding
Hydrogen bonds, a particularly strong type of dipole-dipole interaction, exert a profound influence on boiling points. These bonds form when a hydrogen atom is bonded to a highly electronegative atom such as oxygen, nitrogen, or fluorine. The resulting partial positive charge on the hydrogen atom allows it to interact strongly with the lone pair of electrons on another electronegative atom.
Water (H₂O) serves as a quintessential example. Its extensive network of hydrogen bonds is responsible for its relatively high boiling point (100°C) compared to other molecules of similar molecular weight.
Similarly, alcohols like ethanol (C₂H₅OH) exhibit elevated boiling points due to hydrogen bonding between the hydroxyl (OH) groups of adjacent molecules. The energy required to overcome these strong intermolecular forces directly contributes to the higher boiling point.
Molecular Weight (Molar Mass) and Boiling Point Trends
The molecular weight, or molar mass, of a substance also plays a significant role in determining its boiling point. Generally, for substances with similar types of intermolecular forces, boiling point increases with increasing molecular weight. This correlation arises because larger molecules have a greater surface area, leading to stronger London Dispersion Forces (LDFs).
Consider the series of alkanes: methane (CH₄), ethane (C₂H₆), propane (C₃H₈), and butane (C₄H₁₀). As the carbon chain length increases, the molecular weight increases, and consequently, the boiling point rises. Methane, being the lightest, has the lowest boiling point, while butane has a considerably higher boiling point due to its larger size and greater intermolecular attraction.
This trend highlights the cumulative effect of numerous, albeit weak, LDFs contributing to the overall intermolecular forces, thereby raising the energy needed to overcome these forces and induce boiling.
The Impact of Pressure on Boiling
Boiling is inherently tied to the surrounding pressure. A liquid boils when its vapor pressure equals the external pressure exerted upon it. Consequently, changes in pressure directly affect the boiling point. Lowering the external pressure reduces the temperature required for the liquid's vapor pressure to match, causing it to boil at a lower temperature.
This principle is evident when observing boiling points at different altitudes. At higher altitudes, where atmospheric pressure is lower, water boils at a temperature below 100°C. This explains why cooking times are often longer at high elevations.
Conversely, increasing the pressure elevates the boiling point. Pressure cookers exploit this phenomenon. By increasing the pressure inside the cooker, the boiling point of water is raised, allowing food to cook at a higher temperature and thus more quickly.
Impurities: Disrupting the Boiling Equilibrium
The presence of impurities can significantly alter a substance's boiling point. This effect is primarily governed by colligative properties, which are properties of solutions that depend on the concentration of solute particles rather than their chemical identity.
Impurities generally lower the vapor pressure of a liquid. To reach the point where the vapor pressure equals the external pressure (i.e., the boiling point), a higher temperature is required. This phenomenon is known as boiling point elevation.
Solutions and Boiling Point Elevation
Solutions, such as saltwater or sugar solutions, exhibit boiling point elevation compared to their pure solvents. The extent of the boiling point elevation is proportional to the concentration of the solute particles in the solution, as dictated by the colligative properties.
For instance, adding salt (NaCl) to water increases the boiling point of the solution above 100°C. The sodium and chloride ions disrupt the intermolecular forces of the water, requiring more energy to transition into the gaseous phase. Similarly, dissolving sugar in water elevates the boiling point, although to a different extent depending on the concentration of the sugar.
The magnitude of boiling point elevation is quantitatively described by the equation ΔTb = Kb m i, where ΔTb is the boiling point elevation, Kb is the ebullioscopic constant (a property of the solvent), m is the molality of the solution, and i is the van't Hoff factor (number of particles the solute dissociates into).
Substances Under the Microscope: Exploring Boiling Points Across Different Classes of Compounds
Having established the fundamental factors governing boiling points, it becomes instructive to examine specific classes of chemical compounds. This allows for a more nuanced understanding of how molecular structure and intermolecular forces interplay to determine this critical physical property. By comparing and contrasting different compound classes, we can gain predictive insights into their boiling point behaviors.
Alcohols: The Influence of Hydrogen Bonding and Molecular Weight
Alcohols, characterized by the presence of a hydroxyl (-OH) group, exhibit boiling points that are significantly elevated compared to alkanes of comparable molecular weight.
This disparity is primarily attributed to the capacity of alcohols to engage in strong intermolecular hydrogen bonding. The hydrogen atom, bonded to the highly electronegative oxygen atom, creates a dipole moment that facilitates attraction to other alcohol molecules.
Comparing Methanol and Ethanol
A comparison between methanol (CH3OH) and ethanol (CH3CH2OH) demonstrates the combined influence of hydrogen bonding and molecular weight.
Ethanol, possessing a slightly higher molecular weight and a longer carbon chain, exhibits a higher boiling point (78.37 °C) than methanol (64.7 °C). This highlights the contribution of increased London Dispersion Forces (LDFs) arising from the larger molecular surface area, in addition to the consistent presence of hydrogen bonding.
Alkanes: London Dispersion Forces and Chain Length
Alkanes, composed solely of carbon and hydrogen atoms, are nonpolar molecules. Their intermolecular interactions are primarily governed by London Dispersion Forces (LDFs), also known as Van der Waals forces.
These forces arise from temporary, instantaneous dipoles created by the movement of electrons within the molecule.
The Impact of Carbon Chain Length on Boiling Point
As the carbon chain length of an alkane increases, so does its molecular surface area, leading to a corresponding increase in LDFs.
Consequently, the boiling point increases with increasing chain length. For example, methane (CH4), the simplest alkane, is a gas at room temperature, while butane (C4H10) has a considerably higher boiling point, although still relatively low.
Ethers: A Contrast to Alcohols
Ethers, characterized by an oxygen atom bonded to two alkyl groups (R-O-R'), exhibit lower boiling points compared to alcohols with similar molecular weights.
This difference stems from the reduced capacity of ethers to engage in extensive hydrogen bonding. While the oxygen atom in ethers can act as a hydrogen bond acceptor, it lacks a hydrogen atom directly bonded to it, preventing it from acting as a hydrogen bond donor.
Diethyl Ether vs. Butanol
Consider diethyl ether (CH3CH2OCH2CH3) and butanol (CH3CH2CH2CH2OH). Both have comparable molecular weights, but butanol, an alcohol, has a significantly higher boiling point due to its ability to form strong hydrogen bonds.
Diethyl ether relies primarily on weaker dipole-dipole interactions and LDFs.
Ionic Compounds: Strong Electrostatic Interactions
Ionic compounds, such as sodium chloride (NaCl) and potassium chloride (KCl), possess exceptionally high boiling points. This characteristic stems from the strong electrostatic interactions between oppositely charged ions in the crystal lattice.
These interactions, also referred to as ionic bonds, require substantial energy to overcome, resulting in a high transition temperature from the liquid to the gaseous phase.
Compared to molecular compounds, where intermolecular forces are significantly weaker, ionic compounds exhibit boiling points that are often hundreds or even thousands of degrees Celsius higher.
Organic Solvents: Structure, IMFs, and Boiling Point
The realm of organic solvents presents a diverse landscape of boiling points, each influenced by the intricate interplay of molecular structure and intermolecular forces.
Acetone, a common solvent with a carbonyl group, exhibits dipole-dipole interactions, leading to a moderate boiling point. Toluene, an aromatic hydrocarbon, relies primarily on LDFs and has a higher boiling point than similarly sized alkanes due to the polarizability of its aromatic ring.
The choice of organic solvent in various applications often hinges on its boiling point, influencing factors such as evaporation rate and suitability for specific chemical reactions.
Azeotropes: Mixtures with Constant Boiling Points
Azeotropes are mixtures of two or more liquids that exhibit a constant boiling point and composition, regardless of the extent of distillation. This phenomenon arises from intermolecular interactions that either increase or decrease the vapor pressure of the mixture relative to its individual components.
Azeotropes pose a significant challenge in separation processes, as conventional distillation techniques are incapable of separating the mixture beyond the azeotropic composition. Ethanol and water form a well-known azeotrope.
Water: A Benchmark Solvent
Water (H2O) serves as a ubiquitous solvent and a benchmark for understanding boiling point phenomena. Its relatively high boiling point (100 °C) for its molecular weight is a direct consequence of its exceptional ability to form strong hydrogen bonds.
Each water molecule can participate in up to four hydrogen bonds with neighboring water molecules, creating a cohesive network that requires significant energy to disrupt during vaporization. Water's properties as a solvent is influenced by its boiling point.
Tools of the Trade: Techniques and Equipment for Boiling Point Determination
Having established the fundamental factors governing boiling points, it becomes instructive to examine specific classes of chemical compounds. This allows for a more nuanced understanding of how molecular structure and intermolecular forces interplay to predict and manipulate boiling points in practical applications. Measuring boiling points accurately often relies on specialized equipment and techniques. Understanding these tools is crucial for researchers and practitioners alike.
Distillation Techniques: Separating Mixtures by Boiling Point
Distillation stands as a cornerstone technique in chemistry, leveraging boiling point differences to separate liquid mixtures. The fundamental principle involves heating the mixture to selectively vaporize components based on their boiling points.
The vapor is then cooled and condensed, allowing for the isolation of individual compounds.
Simple distillation is effective for separating liquids with significantly different boiling points.
Fractional distillation, employing a fractionating column, is essential for separating mixtures with closer boiling points, achieving higher purity through repeated vaporization and condensation cycles.
Precision Thermometry: Measuring Boiling Points Accurately
Accurate temperature measurement is paramount in determining boiling points. Precision thermometers, often calibrated against known standards, are employed to ensure reliable data.
These thermometers may utilize various sensing mechanisms, including resistance temperature detectors (RTDs) and thermocouples. The choice depends on the temperature range and accuracy requirements of the experiment.
Digital thermometers offer enhanced precision and ease of reading, often incorporating data logging capabilities for comprehensive analysis.
Barometric Pressure Corrections: Accounting for Altitude
Boiling point is intrinsically linked to atmospheric pressure. As pressure decreases, the boiling point of a liquid also decreases.
Therefore, it is essential to use a barometer to measure atmospheric pressure during boiling point determinations. This allows for corrections to be applied to the observed boiling point, enabling accurate comparisons to literature values obtained at standard pressure (typically 760 mmHg or 1 atm).
Correction factors or nomographs are often used to adjust the boiling point based on the measured pressure.
Reflux Apparatus: Maintaining Constant Boiling
In chemical reactions, maintaining a constant boiling temperature can be crucial for optimal reaction rates and yields.
A reflux apparatus allows for heating a liquid to its boiling point without loss of solvent.
The vapor rises and condenses, flowing back into the reaction flask, creating a continuous cycle of vaporization and condensation. This ensures a consistent reaction temperature while preventing solvent evaporation. This control is key to reactions that require prolonged heating at a specific temperature.
Video: Boiling Point: What Factors Cause Variations?
Frequently Asked Questions About Boiling Point Variations
What role does intermolecular force strength play in determining boiling point?
Stronger intermolecular forces lead to higher boiling points. More energy is needed to overcome these attractive forces and allow molecules to escape into the gas phase. Therefore, when considering what factors can cause a varied boiling point, intermolecular force strength is a primary influence.
How does molecular weight affect the boiling point of a substance?
Generally, boiling point increases with increasing molecular weight. Larger molecules have more electrons, which leads to stronger London dispersion forces, a type of intermolecular force. These increased forces mean what factors can cause a varied boiling point can certainly include the size of the molecule.
Why do branched molecules often have lower boiling points than straight-chain molecules with similar molecular weights?
Branching reduces the surface area available for intermolecular interactions. This weakens the overall strength of London dispersion forces. When considering what factors can cause a varied boiling point, branching and molecular shape should be taken into account alongside size.
Does pressure impact a liquid's boiling point?
Yes, boiling point is directly related to pressure. A higher pressure requires more energy to reach the vapor pressure needed for boiling, thus increasing the boiling point. When considering what factors can cause a varied boiling point, the external atmospheric pressure plays a significant role.
So, the next time you're watching water boil, remember it's not as simple as just reaching 100°C! Pressure, impurities, and even the container you're using can all play a role. Keep these factors in mind – they're the key to understanding what factors can cause a varied boiling point!