O+ vs O- Blood: Differences, Risks, Why It Matters
Understanding the nuances of blood types is critical in healthcare, particularly when considering transfusions and potential health risks. The American Red Cross emphasizes the importance of knowing your blood type because of the implications in emergencies. A key distinction lies in the Rhesus (Rh) factor, a feature that determines whether a blood type is positive (+) or negative (-), directly impacting which blood types can be safely received during transfusions; for example, individuals with O- blood are often called universal donors, as their blood lacks A, B, and Rh antigens, making it compatible with many, but understanding the differences between O+ vs O- blood is vital, especially when considering potential complications during pregnancy, where Rh incompatibility can pose risks to the fetus. Blood donation centers rely on precise blood typing to ensure compatibility, underscoring the need for widespread awareness of blood type characteristics and their impact on medical treatments.
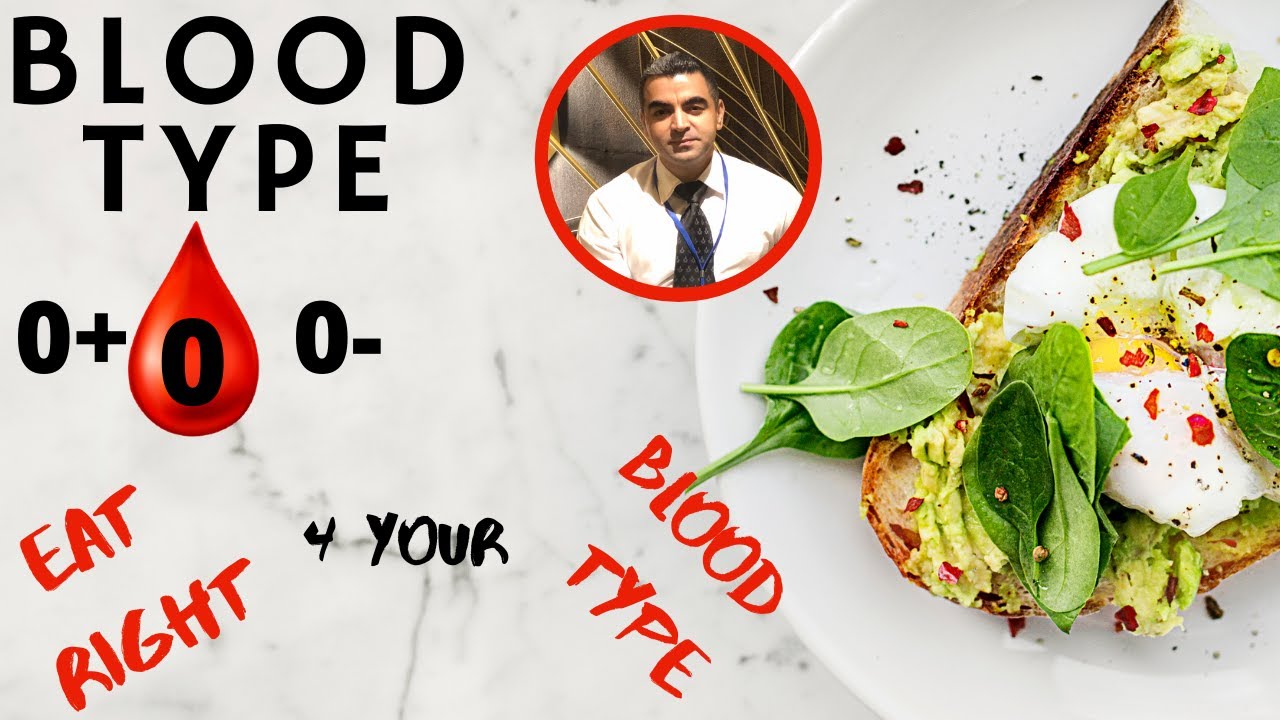
Image taken from the YouTube channel Fit Family Tv , from the video titled The Blood Type Diet || Blood Type "O" ( O+ & O- ) .
The Lifesaving Science of Blood Groups: A Foundation for Modern Medicine
Blood groups are a cornerstone of modern medicine, playing an indispensable role in procedures ranging from routine blood transfusions to the management of complex pregnancies. Their fundamental significance lies in the ability to determine the compatibility, or incompatibility, between individuals' blood, directly impacting patient safety and treatment efficacy.
The Critical Role of Blood Groups
Blood groups are classifications of blood based on the presence or absence of inherited antigenic substances on the surface of red blood cells. These antigens can trigger an immune response if they are foreign to the body. This is why understanding blood types is so vital.
In transfusion medicine, knowledge of blood groups ensures that patients receive blood that their immune system will not reject. Similarly, in pregnancy, particularly when the mother is Rh-negative and the fetus is Rh-positive, awareness of blood groups is crucial for preventing Hemolytic Disease of the Fetus and Newborn (HDFN).
The complexity of blood group systems necessitates rigorous testing and a deep understanding of their implications. Ensuring blood compatibility minimizes the risk of adverse reactions, such as acute hemolytic transfusion reactions, which can be life-threatening.
Preventing Adverse Reactions: A Matter of Life and Death
A comprehensive understanding of blood group systems is not merely academic; it's a practical necessity. Adverse reactions to incompatible blood can range from mild fever and chills to severe complications like kidney failure or disseminated intravascular coagulation (DIC).
Therefore, accurate blood typing and crossmatching are paramount. These procedures identify potential incompatibilities before a transfusion, allowing medical professionals to select blood products that are safe and appropriate for each patient.
In obstetric care, identifying Rh-negative mothers and administering Rh immunoglobulin (RhoGAM) prevents Rh sensitization. This action protects future pregnancies from the risk of HDFN. These preventative measures are predicated on a solid grasp of blood group genetics and immunology.
A Brief History of Blood Group Discovery
The discovery of blood groups at the beginning of the 20th century revolutionized medical practice. Prior to this understanding, blood transfusions were often a dangerous and unpredictable undertaking.
Karl Landsteiner's identification of the ABO blood group system in 1901 marked a watershed moment. His work not only explained why some transfusions were successful while others were fatal, but also laid the groundwork for safe transfusion practices.
Later, the discovery of the Rh factor by Landsteiner and Wiener in the 1940s further refined our understanding of blood compatibility and its implications for pregnancy. The historical evolution of blood group knowledge underscores the ongoing refinement and increased sophistication within the field, improving outcomes for patients worldwide.
Decoding Blood Group Systems: ABO and Rh Factor
Following the introduction to blood groups, it's crucial to understand the specific systems that define blood compatibility. Among the many blood group systems identified, the ABO and Rh (Rhesus) systems are the most clinically significant.
These systems dictate transfusion compatibility and play a crucial role in preventing Hemolytic Disease of the Fetus and Newborn (HDFN).
This section will explore the intricacies of these two primary systems, examining their antigens, antibodies, genetic underpinnings, and global distribution.
The ABO Blood Group System
The ABO blood group system, discovered by Karl Landsteiner in the early 1900s, is based on the presence or absence of two antigens – A and B – on the surface of red blood cells. Individuals are classified into one of four blood types: A, B, AB, or O, depending on which antigens they possess.
Antigens and Antibodies
Type A individuals have A antigens on their red blood cells and anti-B antibodies in their plasma.
Conversely, Type B individuals have B antigens and anti-A antibodies.
Type AB individuals have both A and B antigens and neither anti-A nor anti-B antibodies.
Type O individuals have neither A nor B antigens but possess both anti-A and anti-B antibodies.
These antibodies are naturally occurring, meaning they are present even without prior exposure to foreign blood.
Genetics of ABO Blood Groups
The ABO blood group is determined by a single gene with three common alleles: A, B, and O. The A and B alleles are codominant, meaning that if both are present, both antigens are expressed (resulting in blood type AB).
The O allele is recessive, meaning that an individual must inherit two copies of the O allele to have blood type O.
This genetic inheritance pattern results in various possible genotypes and corresponding phenotypes (blood types).
For example, an individual with the genotype AA or AO will have blood type A, while an individual with the genotype BB or BO will have blood type B.
Global Distribution and Evolutionary Considerations
The distribution of ABO blood types varies significantly across different populations worldwide.
For example, blood type O is more prevalent in indigenous populations of South America, while blood type B is more common in parts of Asia.
These variations are thought to be due to a combination of factors, including founder effects, genetic drift, and natural selection.
Some research suggests that certain blood types may offer protection against specific infectious diseases.
For instance, individuals with blood type O may be more susceptible to severe cholera infections but less susceptible to severe malaria. These selective pressures could have contributed to the observed global distribution of ABO blood types.
The Rh Factor (Rhesus Factor)
The Rh factor, also known as the Rhesus factor, is another crucial blood group system.
It is defined by the presence or absence of the D antigen on red blood cells.
Individuals who have the D antigen are considered Rh-positive (Rh+), while those who lack the D antigen are considered Rh-negative (Rh-).
Clinical Significance: Rh Sensitization and HDFN
The Rh factor is particularly important in the context of pregnancy.
If an Rh-negative woman carries an Rh-positive fetus, she can become sensitized to the Rh antigen if fetal blood enters her circulation. This can occur during delivery, miscarriage, or other invasive procedures.
Once sensitized, the mother's immune system will produce anti-RhD antibodies.
In subsequent pregnancies with an Rh-positive fetus, these antibodies can cross the placenta and attack the fetal red blood cells, leading to Hemolytic Disease of the Fetus and Newborn (HDFN).
HDFN can cause severe anemia, jaundice, and even fetal death.
Genetic Inheritance of the Rh Factor
The Rh factor is primarily determined by the RHD gene, which encodes the D antigen.
The presence of the RHD gene indicates Rh-positive blood type, while the absence of the gene (often due to a deletion) indicates Rh-negative blood type.
The inheritance pattern is relatively straightforward: Rh-positive individuals have at least one copy of the RHD gene, while Rh-negative individuals have two copies of the non-functional RHD gene (or a deletion).
Understanding the genetic inheritance of the Rh factor is critical for predicting the risk of Rh incompatibility in pregnancies.
Key Players: Antigens, Antibodies, and Blood Cells in Action
Following the introduction to blood groups, it's crucial to understand the specific systems that define blood compatibility. Among the many blood group systems identified, the ABO and Rh (Rhesus) systems are the most clinically significant.
These systems dictate transfusion compatibility and play a crucial role in preventing adverse reactions. To fully grasp their implications, it's essential to delve into the biological components and processes involved, specifically the roles of antigens, antibodies, and blood cells.
Understanding Antigens: The Identity Markers
Antigens are substances, usually proteins or carbohydrates, found on the surface of red blood cells. They act as identity markers, distinguishing one blood group from another.
In the ABO system, the key antigens are A and B. An individual with type A blood has A antigens on their red blood cells, while someone with type B blood has B antigens. Individuals with type AB blood have both A and B antigens, and those with type O blood have neither.
The presence or absence of these antigens is genetically determined, making them a reliable identifier of a person's blood type.
Antibodies: The Immune System's Responders
Antibodies are proteins produced by the immune system to recognize and bind to foreign substances, including antigens not found on an individual's own red blood cells.
Individuals with type A blood have anti-B antibodies in their plasma, meaning their immune system will attack any red blood cells with B antigens. Conversely, those with type B blood have anti-A antibodies.
Type O individuals have both anti-A and anti-B antibodies, making them particularly susceptible to reactions from type A, B, or AB blood. Type AB individuals, on the other hand, have neither anti-A nor anti-B antibodies.
This is a critical factor in determining blood transfusion compatibility.
Red Blood Cells (Erythrocytes): The Oxygen Carriers
Red blood cells, also known as erythrocytes, are the most abundant cells in the blood and are responsible for transporting oxygen from the lungs to the body's tissues.
Their surface is covered with antigens that define blood groups, making them the primary target of antibody-mediated immune responses.
The interaction between antigens on red blood cells and antibodies in the plasma determines whether a blood transfusion is compatible or incompatible.
Plasma: The Antibody-Rich Medium
Plasma is the liquid component of blood, making up about 55% of its total volume. It contains water, proteins, electrolytes, and other substances, including antibodies.
The antibodies present in plasma play a crucial role in determining blood compatibility. As mentioned earlier, individuals with type A blood have anti-B antibodies in their plasma, and so on.
During a blood transfusion, it's essential to ensure that the recipient's plasma does not contain antibodies that will react with the donor's red blood cells.
Agglutination: The Clumping Reaction
Agglutination occurs when antibodies bind to antigens on red blood cells, causing the cells to clump together. This clumping can obstruct blood flow and lead to serious complications.
For example, if a person with type A blood receives type B blood, the anti-B antibodies in their plasma will bind to the B antigens on the donor's red blood cells, causing agglutination.
This is why blood typing and crossmatching are essential before any blood transfusion to prevent such reactions.
Hemolysis: The Destruction of Red Blood Cells
Hemolysis is the destruction of red blood cells. It can occur as a result of an antibody-antigen reaction, where the immune system attacks and destroys incompatible red blood cells.
This process releases hemoglobin into the bloodstream, which can damage organs and lead to severe complications. Hemolysis is a critical concern in both transfusion reactions and Hemolytic Disease of the Fetus and Newborn (HDFN).
In HDFN, maternal antibodies attack fetal red blood cells, leading to hemolysis and potentially life-threatening consequences for the fetus.
The Interplay: Compatibility and Incompatibility
The interaction between antigens, antibodies, and blood cells determines blood compatibility and incompatibility.
When compatible blood is transfused, the recipient's immune system does not recognize the donor's red blood cells as foreign, and no immune response is triggered.
However, when incompatible blood is transfused, the recipient's antibodies bind to the donor's red blood cells, leading to agglutination and hemolysis. This can result in a range of adverse reactions, from mild fever and chills to life-threatening organ damage.
Understanding these key players and their interactions is paramount to ensuring safe blood transfusions and preventing adverse outcomes in conditions like HDFN. Careful blood typing, crossmatching, and monitoring are essential to safeguard patient health.
Ensuring Safe Transfusions: Blood Compatibility and Testing
Following the introduction to blood groups, it's crucial to understand the specific systems that define blood compatibility. Among the many blood group systems identified, the ABO and Rh (Rhesus) systems are the most clinically significant.
These systems dictate transfusion compatibility, influencing procedures from initial blood typing to the final stages of patient monitoring.
The Cornerstones of Transfusion Safety
Blood Typing: Identifying Blood Groups
Blood typing is the foundational step in ensuring safe blood transfusions.
This process involves laboratory techniques used to identify an individual's ABO and Rh blood group.
By determining the presence or absence of specific antigens on red blood cells, healthcare professionals can classify blood into A, B, AB, or O, and Rh-positive or Rh-negative.
Crossmatching: Verifying Compatibility
Crossmatching is a critical step performed after blood typing to confirm compatibility between the donor and recipient blood.
This test involves mixing a sample of the recipient's plasma with the donor's red blood cells.
If no agglutination (clumping) occurs, the blood is considered compatible and safe for transfusion.
This step is vital for preventing potentially life-threatening transfusion reactions.
The Transfusion Process: A Step-by-Step Approach
Blood transfusion involves the controlled administration of blood or blood components to a patient.
Before initiating a transfusion, patient identification must be verified meticulously to prevent errors.
During the transfusion, vital signs are closely monitored to detect any early signs of a reaction.
Post-transfusion monitoring is essential to ensure the patient's well-being and assess the effectiveness of the transfusion.
Historical Perspectives: Universal Donors and Recipients Reconsidered
The Myth of the Universal Donor
Historically, O-negative blood was considered the "universal donor" because it lacks A, B, and Rh antigens.
While O-negative red blood cells can be transfused to most individuals in emergencies, this practice is not without limitations.
O-negative blood still contains antibodies that can cause reactions in some patients, making it essential to reserve O-negative blood for specific situations and prioritize type-specific transfusions when possible.
The Misconception of the Universal Recipient
Conversely, AB-positive blood was once regarded as the "universal recipient" due to the absence of anti-A and anti-B antibodies.
However, AB-positive individuals can still experience adverse reactions if transfused with blood containing incompatible antibodies.
The concept of a universal recipient is now considered inaccurate, highlighting the importance of crossmatching even in AB-positive patients.
Tailoring Transfusions: Blood Components and Their Uses
Modern transfusion practices utilize specific blood components to address particular patient needs.
Red blood cells are transfused to treat anemia and improve oxygen-carrying capacity.
Plasma is administered to address clotting factor deficiencies.
Platelets are given to prevent or treat bleeding disorders.
This component-based approach minimizes unnecessary exposure to blood products and optimizes therapeutic outcomes.
Recognizing and Managing Transfusion Reactions
Transfusion reactions are adverse events that can occur during or after a blood transfusion.
Symptoms can range from mild (fever, chills, hives) to severe (hemolytic reactions, anaphylaxis).
Prompt recognition and management of transfusion reactions are crucial to prevent serious complications.
Healthcare providers must be trained to identify the signs and symptoms of transfusion reactions and initiate appropriate interventions, which may include stopping the transfusion, administering medications, and providing supportive care.
HDFN: Understanding and Preventing Hemolytic Disease of the Fetus and Newborn
Following the critical safety measures in blood transfusions, understanding the complexities of blood group incompatibility during pregnancy is equally paramount. Hemolytic Disease of the Fetus and Newborn (HDFN) presents a significant challenge in maternal-fetal medicine.
This condition arises from an immunological conflict between mother and fetus, demanding comprehensive knowledge for effective management and prevention.
The Pathophysiology of HDFN: Maternal-Fetal Immunological Conflict
HDFN is primarily triggered by maternal antibodies targeting fetal red blood cells (RBCs). These antibodies, typically IgG, cross the placenta and bind to fetal RBCs expressing the corresponding antigen. The most common scenario involves RhD incompatibility, where an Rh-negative mother carries an Rh-positive fetus. However, incompatibilities involving other blood group systems, such as ABO, Kell, and Duffy, can also lead to HDFN, albeit less frequently and often with milder consequences.
The binding of maternal antibodies to fetal RBCs initiates a cascade of events. Sensitized fetal RBCs are prematurely destroyed (hemolyzed) by phagocytic cells in the fetal spleen and liver. This accelerated destruction leads to fetal anemia.
Clinical Consequences: A Spectrum of Severity
The clinical consequences of HDFN can range from mild to life-threatening for the fetus and newborn.
Anemia is a central feature, impairing oxygen delivery to fetal tissues. In response to anemia, the fetal bone marrow increases RBC production, sometimes leading to extramedullary hematopoiesis in the liver and spleen. This can cause organomegaly (enlargement of organs).
Jaundice develops due to the buildup of bilirubin, a byproduct of hemoglobin breakdown, after the destruction of RBCs. While the maternal liver usually clears bilirubin during pregnancy, the newborn liver may be unable to process the excessive bilirubin load efficiently, leading to hyperbilirubinemia and potential neurological damage (kernicterus).
Hydrops Fetalis represents the most severe form of HDFN. It is characterized by generalized edema, ascites (fluid accumulation in the abdominal cavity), and pleural effusion (fluid around the lungs). Hydrops fetalis results from severe anemia, impaired cardiac function, and decreased oncotic pressure. It often leads to fetal death if untreated.
In less severe cases, the infant may only experience mild anemia and jaundice requiring phototherapy. In the most severe situations, miscarriage or stillbirth can occur.
Rh Sensitization: The Development of Maternal Antibodies
Rh sensitization is the process by which an Rh-negative individual develops anti-RhD antibodies after exposure to Rh-positive blood.
This exposure can occur through various mechanisms, most commonly during pregnancy when fetal Rh-positive RBCs enter the maternal circulation. This transplacental passage of fetal blood can occur during delivery, spontaneous or induced abortion, ectopic pregnancy, amniocentesis, or abdominal trauma.
Once sensitized, the Rh-negative mother will produce anti-RhD antibodies. These antibodies pose a threat in subsequent pregnancies with Rh-positive fetuses.
Sensitization can also occur through mismatched blood transfusions, though this is far less common today due to rigorous blood typing and crossmatching procedures.
ABO Incompatibility: A Unique Consideration
While Rh incompatibility is often highlighted, ABO incompatibility also contributes to HDFN.
Unlike Rh disease, ABO incompatibility can occur in the first pregnancy. This is because most individuals possess naturally occurring anti-A and anti-B antibodies.
The most common scenario involves a mother with blood type O carrying a fetus with blood type A or B. Maternal anti-A or anti-B IgG antibodies can cross the placenta and cause hemolysis of fetal RBCs. However, ABO incompatibility usually results in a milder form of HDFN compared to Rh incompatibility. This is because ABO antigens are less densely expressed on fetal RBCs, and anti-A and anti-B antibodies are often IgM (which does not cross the placenta readily) or lower affinity IgG.
Prevention is Paramount: The Role of Rhogam
The introduction of Rho(D) immune globulin (RhoGAM) has dramatically reduced the incidence of Rh-related HDFN.
RhoGAM contains anti-RhD antibodies that bind to any fetal Rh-positive RBCs in the maternal circulation, preventing the mother’s immune system from recognizing them and developing her own anti-RhD antibodies.
RhoGAM is typically administered to Rh-negative pregnant women at 28 weeks of gestation and again within 72 hours after delivery of an Rh-positive infant. It is also given after any event that could potentially cause fetal-maternal hemorrhage, such as amniocentesis or abdominal trauma.
HDFN remains a complex and potentially serious condition. A thorough understanding of its pathophysiology, clinical consequences, and preventative strategies is essential for all healthcare professionals involved in maternal-fetal care. Through careful monitoring, timely interventions, and the judicious use of RhoGAM, we can significantly reduce the morbidity and mortality associated with HDFN and ensure the best possible outcomes for both mother and child.
Diagnosis: Identifying Risks and Detecting HDFN
Following the critical safety measures in blood transfusions, understanding the complexities of blood group incompatibility during pregnancy is equally paramount. Hemolytic Disease of the Fetus and Newborn (HDFN) presents a significant challenge in maternal-fetal medicine. Effective diagnosis is crucial for timely intervention and improving outcomes. This section outlines the array of diagnostic tools employed to identify risks and detect HDFN, both during pregnancy and after birth.
Prenatal Screening and Risk Assessment
The cornerstone of managing HDFN risk lies in comprehensive prenatal screening. This involves a series of blood tests conducted on pregnant women to determine their blood type and screen for the presence of atypical antibodies.
- Blood Typing and Antibody Screening: At the initial prenatal visit, all pregnant women undergo blood typing to determine their ABO and RhD status. RhD-negative women are of particular concern, as they are at risk of RhD alloimmunization if carrying an RhD-positive fetus. Antibody screening identifies the presence of any atypical antibodies in the maternal serum, including anti-RhD or other antibodies capable of causing HDFN. If atypical antibodies are detected, their specificity and titer are determined to assess the risk to the fetus.
Invasive vs. Non-Invasive Diagnostic Techniques
The diagnosis of HDFN may involve both non-invasive and invasive techniques, each with its own advantages and risks. Non-invasive methods are preferred initially, reserving invasive procedures for cases where further evaluation is necessary.
Non-Invasive Doppler Ultrasound
Doppler ultrasound is a non-invasive technique used to assess fetal anemia by measuring blood flow velocity in the middle cerebral artery (MCA).
- Increased MCA blood flow velocity is indicative of fetal anemia, as the fetus compensates by increasing cardiac output. Doppler ultrasound is a valuable tool for monitoring fetal status and guiding decisions regarding further intervention. This method has largely replaced invasive methods as a primary means of assessing fetal anemia.
Invasive Procedures: Amniocentesis and Cordocentesis
Invasive procedures such as amniocentesis and cordocentesis are reserved for cases where further evaluation is needed after initial risk assessment.
-
Amniocentesis: Amniocentesis involves obtaining a sample of amniotic fluid. While once used to measure bilirubin levels, its role has diminished. It is now primarily used to assess fetal lung maturity in cases of impending preterm delivery due to HDFN.
-
Cordocentesis (PUBS): Cordocentesis, also known as Percutaneous Umbilical Blood Sampling (PUBS), involves obtaining a fetal blood sample directly from the umbilical cord. This procedure allows for direct assessment of fetal hematocrit and bilirubin levels.
Cordocentesis carries a higher risk of complications, such as fetal bleeding or pregnancy loss. Therefore, it is reserved for severe cases where precise assessment of fetal status is essential for guiding treatment decisions.
Postnatal Diagnosis: Assessing the Newborn
Postnatal diagnosis of HDFN focuses on assessing the newborn for signs of anemia, jaundice, and other complications. The direct Coombs test is a crucial diagnostic tool in this setting.
Direct and Indirect Coombs Tests
The Coombs test, also known as the antiglobulin test, is used to detect antibodies that are bound to the surface of red blood cells.
-
The direct Coombs test is performed on the newborn's blood to detect antibodies already attached to the infant's red blood cells.
-
The indirect Coombs test is performed on the maternal serum to detect unbound antibodies that are capable of reacting with red blood cells.
A positive direct Coombs test in the newborn indicates that maternal antibodies have crossed the placenta and are attacking the infant's red blood cells, confirming the diagnosis of HDFN.
Treatment Strategies: Managing HDFN and Preventing Complications
Following the critical safety measures in blood transfusions, understanding the complexities of blood group incompatibility during pregnancy is equally paramount. Hemolytic Disease of the Fetus and Newborn (HDFN) presents a significant challenge in maternal-fetal medicine. Effective diagnosis is crucial; however, the management and prevention of HDFN through timely and appropriate interventions are equally important to ensure favorable outcomes for both mother and child.
Preventing Rh Sensitization: The Role of Rho(D) Immune Globulin
Rho(D) Immune Globulin (RhoGAM) represents a cornerstone in preventing Rh sensitization in Rh-negative pregnant women. This prophylactic measure involves administering anti-RhD antibodies to the mother, effectively preventing her immune system from recognizing and responding to Rh-positive fetal red blood cells that may have entered her circulation.
The standard protocol includes administering RhoGAM at around 28 weeks of gestation and again within 72 hours after delivery if the newborn is Rh-positive. Furthermore, it is administered after any event with the potential for fetal-maternal hemorrhage, such as miscarriage, ectopic pregnancy, or invasive procedures.
The effectiveness of RhoGAM has dramatically reduced the incidence of Rh sensitization and subsequent HDFN, highlighting its crucial role in prenatal care. Proper administration and adherence to recommended guidelines are essential to maximize its protective benefits.
Managing Hyperbilirubinemia: Phototherapy and IVIG
Once HDFN is diagnosed, the primary focus shifts to managing the resulting hyperbilirubinemia and anemia in the newborn.
Phototherapy
Phototherapy is a widely used and effective treatment for neonatal jaundice. It involves exposing the newborn's skin to specific wavelengths of light, which convert bilirubin into a water-soluble form that can be easily excreted in the urine and stool.
This non-invasive treatment helps to reduce bilirubin levels and prevent the development of kernicterus, a rare but devastating neurological condition caused by bilirubin accumulation in the brain. Careful monitoring of bilirubin levels and appropriate adjustments to phototherapy intensity are essential to optimize treatment outcomes.
Intravenous Immunoglobulin (IVIG)
In some cases of HDFN, Intravenous Immunoglobulin (IVIG) may be administered to reduce the levels of maternal antibodies attacking the newborn's red blood cells.
IVIG works by saturating the Fc receptors on macrophages, thereby reducing the rate of hemolysis. It is particularly useful in cases where phototherapy alone is insufficient to control hyperbilirubinemia or when anemia is significant.
Addressing Anemia: Intrauterine and Exchange Transfusions
Severe cases of HDFN can lead to significant fetal or neonatal anemia, requiring more invasive interventions such as intrauterine and exchange transfusions.
Intrauterine Transfusion (IUT)
Intrauterine Transfusion (IUT) is a procedure performed when fetal anemia is detected before birth. Under ultrasound guidance, compatible red blood cells are transfused directly into the fetal circulation, correcting the anemia and supporting fetal well-being until delivery. IUT is a complex procedure that requires expertise and careful monitoring but can significantly improve outcomes in severe cases of HDFN.
Exchange Transfusion (ET)
Exchange Transfusion (ET) is performed after birth to rapidly remove bilirubin and maternal antibodies from the newborn's circulation. This procedure involves gradually replacing the newborn's blood with donor blood in small aliquots, effectively diluting the bilirubin and antibody concentrations. ET is typically reserved for severe cases of hyperbilirubinemia or anemia that are not responsive to other treatments.
Comparing Treatment Strategies: A Holistic Approach
The management of HDFN requires a comprehensive approach, combining preventive measures like RhoGAM with targeted interventions such as phototherapy, IVIG, and transfusions. The choice of treatment depends on the severity of the condition and the gestational age of the fetus or newborn.
Early detection and timely intervention are crucial for minimizing the risks associated with HDFN and ensuring the best possible outcomes for both mother and child. A multidisciplinary team involving obstetricians, neonatologists, and transfusion medicine specialists is essential for optimal management of these complex cases.
Following the critical safety measures in blood transfusions, understanding the complexities of blood group incompatibility during pregnancy is equally paramount. Hemolytic Disease of the Fetus and Newborn (HDFN) presents a significant challenge in maternal-fetal medicine. Effective diagnosis and treatment strategies can significantly mitigate risks, but the cornerstone of managing blood-related medical needs rests on a solid foundation: a safe and adequate blood supply.
Beyond Transfusions: Blood Donation, Supply, and Safety
The intricacies of blood groups extend beyond transfusion compatibility and managing pregnancy-related complications. The availability of safe blood for all who need it is a critical public health imperative. This relies on voluntary blood donation, efficient blood supply management, and stringent safety measures. Understanding each facet is crucial for ensuring a robust and reliable system.
The Lifeline of Blood Donation
Blood donation forms the basis of a functioning blood supply. Without the altruistic contributions of voluntary donors, the ability to provide life-saving transfusions would be severely compromised. The process involves healthy individuals donating a unit of blood, which is then processed and made available for clinical use.
Encouraging blood donation is a continuous effort. Education campaigns play a vital role in raising awareness about the importance of donation and dispelling common misconceptions. Regular donors are the backbone of a sustainable blood supply.
The donation process itself is carefully regulated to ensure the safety of both the donor and the recipient. Potential donors undergo a screening process to assess their eligibility. This includes a review of their medical history and a physical examination.
Blood Supply: A Complex Logistical Chain
Managing the blood supply is a multifaceted operation. It involves the efficient collection, processing, testing, storage, and distribution of blood products. Effective management is essential to minimize waste and ensure that the right blood type is available when and where it is needed.
Collection and Processing
Blood is collected at donation centers or mobile blood drives. After collection, the blood is processed into its various components: red blood cells, plasma, platelets, and cryoprecipitate. This allows for targeted transfusions, where patients receive only the specific blood component they require.
Storage and Distribution
Proper storage is critical to maintain the viability of blood products. Red blood cells, for example, have a limited shelf life. A sophisticated logistical network is necessary to ensure that blood products are distributed efficiently to hospitals and other healthcare facilities.
Ensuring Blood Safety: A Multi-Layered Approach
Blood safety is of utmost importance. Rigorous testing and screening procedures are implemented to minimize the risk of transmitting infectious diseases through blood transfusions.
Donor Screening
Potential donors are screened for risk factors that may increase their likelihood of carrying infectious agents. This includes questions about their medical history, travel history, and lifestyle.
Blood Testing
All donated blood is tested for a range of infectious diseases, including HIV, hepatitis B, hepatitis C, syphilis, and West Nile virus. Nucleic acid amplification testing (NAT) is often used to detect viral RNA or DNA, providing an early and sensitive indication of infection.
Pathogen Reduction Technologies
Pathogen reduction technologies (PRTs) are increasingly being used to further enhance blood safety. These technologies aim to inactivate or remove pathogens from blood products, reducing the risk of transfusion-transmitted infections.
Blood safety is an ongoing process. New threats and emerging infections require continuous vigilance and adaptation of screening and testing protocols. Collaboration between blood banks, public health agencies, and research institutions is essential to maintaining a safe blood supply.
Video: O+ vs O- Blood: Differences, Risks, Why It Matters
FAQs: O+ vs O- Blood
What's the main difference between O+ and O- blood?
The primary difference between o+ vs o- blood lies in the presence or absence of the Rh factor. O+ blood has the Rh factor (positive), while O- blood lacks it (negative). This single difference impacts who can safely receive a transfusion of each blood type.
Why is O- blood called the "universal donor" and can O+ blood be used for anyone?
O- blood is the universal donor because it lacks A, B, and Rh antigens, meaning it can be transfused to individuals with any blood type without causing a reaction. O+ blood, however, contains the Rh antigen, so it cannot be given to those with O- blood because they will react to the Rh factor.
What are the potential risks of receiving the wrong blood type during a transfusion, especially concerning o+ vs o- blood?
Receiving the wrong blood type can trigger a severe immune reaction. In the context of o+ vs o- blood, an O- individual receiving O+ blood will likely develop antibodies against the Rh factor. This can cause dangerous clumping of blood cells, leading to organ damage and potentially death.
If both parents are O+, what blood type(s) can their children have? Can they have O- blood?
If both parents are O+, their children can be either O+ or O-. The parents must both carry the recessive "O" gene and the Rh-negative gene (hidden by the "+" dominant gene). Therefore, the child can inherit the recessive Rh-negative gene from both parents, resulting in O- blood.
So, there you have it! Hopefully, this clears up some of the confusion around O+ vs O- blood, their key differences, and why knowing your type is actually pretty important. It's not just a fun fact for awkward introductions – it can literally be a lifesaver. Now go forth and spread the knowledge (and maybe consider donating blood while you're at it!).