Bacterial Structure: The Ultimate Guide You NEED to Know
Understanding bacterial structure is fundamental to comprehending microbial life. The cell wall, a key component of bacterial structure, dictates bacterial shape and provides protection against osmotic pressure. Knowledge of this crucial detail is essential for the development of effective antibiotics, a critical area of research currently conducted at institutions like the Centers for Disease Control and Prevention (CDC). Furthermore, advancements in microscopy techniques continue to refine our understanding of bacterial structure, enabling detailed visualization of its intricate components and contributing to breakthroughs in tackling bacterial resistance. The intricate world of bacterial structure ultimately offers a window into understanding how bacteria interact with their environments.
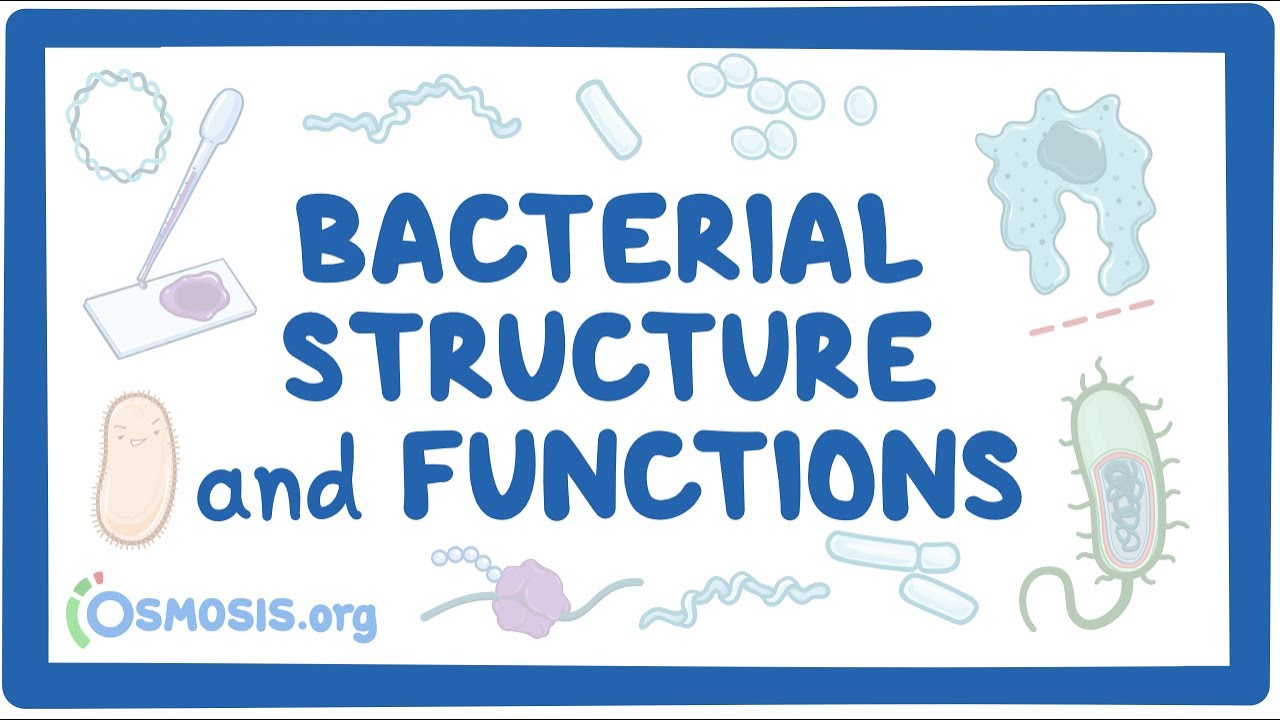
Image taken from the YouTube channel Osmosis from Elsevier , from the video titled Bacterial Structure and Functions .
Bacteria, often unseen and unappreciated, are the cornerstones of life on Earth. These microscopic organisms, classified as prokaryotes, are not just simple entities; they are complex systems with intricate structures that dictate their behavior and interactions within diverse environments.
Their pervasive presence and multifaceted roles underscore the critical importance of comprehending their biology, particularly their structural intricacies. This understanding is not merely academic; it holds the key to unlocking advancements in medicine, environmental science, and various industrial applications.
Bacteria: The Quintessential Prokaryotes
At the heart of understanding bacteria lies the distinction between prokaryotic and eukaryotic cells. Bacteria, as prokaryotes, lack a membrane-bound nucleus and other complex organelles characteristic of eukaryotic cells, such as those found in plants and animals.
Instead, their genetic material resides within the cytoplasm in a region called the nucleoid. This fundamental difference in cellular organization has profound implications for bacterial physiology, reproduction, and response to external stimuli. Understanding this distinction is critical when designing targeted therapeutics.
The Ubiquitous Influence of Bacteria
The influence of bacteria extends to virtually every corner of our planet. In the environment, they are essential for nutrient cycling, decomposition, and maintaining ecological balance.
They play a vital role in the carbon and nitrogen cycles, breaking down organic matter and converting atmospheric nitrogen into usable forms for plants. Within the human body, bacteria form complex communities known as the microbiome, which play a critical role in digestion, immunity, and overall health.
Furthermore, bacteria are indispensable in various industrial processes, including the production of foods (yogurt, cheese), pharmaceuticals (antibiotics, insulin), and biofuels. Their metabolic versatility makes them valuable tools for biotechnology and bioremediation.
The Imperative of Structural Understanding
The study of bacterial structure is far more than an academic pursuit; it is a crucial endeavor with far-reaching implications for human health and well-being.
A thorough understanding of the bacterial cell wall, membrane, and other structural components is essential for developing new antibiotics that can effectively target and disrupt bacterial function.
As antibiotic resistance continues to rise, the need for innovative strategies to combat bacterial infections becomes increasingly urgent. By elucidating the precise mechanisms by which bacteria interact with their environment and cause disease, we can design targeted interventions to prevent and treat infections more effectively. Understanding bacterial structure is also essential for developing effective vaccines and diagnostic tools.
The Bacterial Cell Envelope: A Fortress of Protection
Having established the fundamental differences between bacteria and other life forms, we now turn our attention to the intricate architecture that safeguards these microscopic entities. The bacterial cell envelope, a complex multi-layered structure, is the first line of defense against a constantly changing and often hostile environment. It's a dynamic interface, mediating interactions with the outside world while maintaining the integrity of the cell's interior.
This section will explore the components of this crucial barrier, detailing the structure and function of the cell wall, cell membrane, and capsule. Understanding these components provides insight into how bacteria survive, thrive, and interact with their environment, a crucial understanding for countering harmful pathogens.
Cell Wall: The Defining Armor
The bacterial cell wall is a rigid structure located outside the cell membrane. Its primary function is to provide shape and structural support, protecting the cell from lysis due to internal osmotic pressure. This pressure can be substantial, especially in hypotonic environments where water rushes into the cell. Without the cell wall, the cell would swell and burst.
Peptidoglycan: The Structural Core
Peptidoglycan (also known as murein) is the defining component of the bacterial cell wall, a unique polymer found only in bacteria. This complex mesh-like structure consists of glycan chains composed of alternating N-acetylglucosamine (NAG) and N-acetylmuramic acid (NAM) residues. These chains are cross-linked by short peptides, creating a strong, interconnected network.
The precise structure of peptidoglycan varies slightly between bacterial species, but its essential function remains the same: to provide rigidity and prevent cellular rupture. The integrity of peptidoglycan is critical for bacterial survival, making it a prime target for many antibiotics, such as penicillin and vancomycin.
Gram-Positive vs. Gram-Negative Bacteria: A Key Distinction
Bacteria are broadly classified into two major groups based on their cell wall structure: Gram-positive and Gram-negative. This distinction, revealed by the Gram stain, has profound implications for their susceptibility to antibiotics and their interactions with the host immune system.
Gram-positive bacteria are characterized by a thick layer of peptidoglycan, which can comprise up to 90% of the cell wall. Interwoven within this peptidoglycan layer are teichoic acids, unique polymers that extend throughout the cell wall and are sometimes covalently linked to the cell membrane (lipoteichoic acids). Teichoic acids contribute to the cell wall's negative charge and play roles in cell adhesion and structural integrity.
In contrast, Gram-negative bacteria possess a much thinner peptidoglycan layer (only a few layers thick), located in the periplasmic space between the inner cell membrane and an outer membrane. The outer membrane is a defining feature of Gram-negative bacteria, composed of a unique lipopolysaccharide (LPS) molecule in its outer leaflet.
Lipopolysaccharide (LPS): A Potent Immunogen
Lipopolysaccharide (LPS), also known as endotoxin, is a potent immunostimulant that triggers a strong inflammatory response in animals. LPS is composed of three parts:
- Lipid A, a hydrophobic anchor embedded in the outer membrane
- A core oligosaccharide
- An O-specific polysaccharide (O-antigen)
The O-antigen is highly variable between bacterial strains and contributes to the serological diversity of Gram-negative bacteria. LPS is a critical virulence factor, contributing to the pathogenicity of many Gram-negative infections.
Cell Membrane (Plasma Membrane): The Selective Barrier
Beneath the cell wall lies the cell membrane, also known as the plasma membrane. This vital structure surrounds the cytoplasm and acts as a selective barrier, controlling the movement of substances into and out of the cell.
The cell membrane is composed of a phospholipid bilayer with proteins embedded within it. The phospholipid molecules have a hydrophilic (water-loving) head and a hydrophobic (water-fearing) tail. These molecules arrange themselves into two layers, with the hydrophobic tails facing inward and the hydrophilic heads facing outward, creating a barrier to the movement of polar molecules.
The proteins embedded within the lipid bilayer perform a variety of functions, including:
- Selective permeability: Regulating the passage of specific ions and molecules
- Nutrient transport: Facilitating the uptake of essential nutrients
- Waste removal: Eliminating metabolic byproducts
- Energy generation: Housing proteins involved in respiration and ATP synthesis.
The cell membrane is a dynamic and essential component of the bacterial cell, ensuring its survival and enabling it to interact with its environment in a controlled manner.
Capsule: An Additional Shield
Some bacteria possess an additional layer outside the cell wall called the capsule. This structure is typically composed of polysaccharides (sugar polymers) but can sometimes be made of polypeptides. The capsule is not present in all bacterial species, but when present, it plays a significant role in bacterial virulence and survival.
The capsule's functions include:
- Virulence: Enhancing the bacterium's ability to cause disease.
- Adhesion: Facilitating attachment to host cells or surfaces.
- Protection against phagocytosis: Inhibiting engulfment and destruction by immune cells.
The capsule's sticky consistency allows bacteria to adhere to surfaces, forming biofilms that are resistant to antibiotics and disinfectants. Moreover, the capsule can mask bacterial surface antigens, preventing recognition and attack by the host's immune system. Bacteria with capsules are often more pathogenic than their non-encapsulated counterparts.
Appendages: Tools for Movement and Attachment
Having explored the protective layers that shield bacteria, it's time to consider the structures that enable these microorganisms to interact with their surroundings. The bacterial cell isn't merely a passive entity; it actively navigates its environment, seeking nutrients, evading threats, and colonizing new territories. This dynamic behavior is largely driven by specialized appendages: flagella and pili (also known as fimbriae). These external structures are essential for bacterial motility, adhesion, and ultimately, survival.
Flagella: The Propellers of Bacteria
Flagella are whip-like appendages that provide bacteria with the ability to move, a characteristic known as motility. These complex structures are not simply passive propellers; they are sophisticated molecular machines that enable bacteria to swim through liquids and even move across surfaces.
Structure of the Flagellum
The bacterial flagellum consists of three main parts:
-
Filament: The long, helical filament is the part of the flagellum that extends out from the cell and generates the propulsive force. It is composed of a protein called flagellin, arranged in a helical chain.
-
Hook: The hook is a curved structure that connects the filament to the basal body. It acts as a flexible joint, allowing the filament to rotate freely.
-
Basal Body: The basal body is a complex motor embedded in the cell envelope. It consists of a series of rings and proteins that generate the torque needed to rotate the flagellum.
Mechanism of Movement
Bacterial flagella rotate like propellers, driven by the basal body motor. This motor is powered by the flow of ions (usually protons or sodium ions) across the cell membrane. The direction of rotation determines the bacterium's movement: counterclockwise rotation typically results in forward motion ("running"), while clockwise rotation causes the cell to tumble and change direction.
Chemotaxis: Navigating the Environment
Bacteria can sense chemical gradients in their environment and move towards attractants (e.g., nutrients) and away from repellents (e.g., toxins). This process is called chemotaxis. Bacteria achieve chemotaxis by modulating the frequency of tumbles. If a bacterium is moving in the right direction (towards an attractant), it will tumble less frequently, resulting in longer runs. If it's moving in the wrong direction, it will tumble more frequently, allowing it to reorient itself. This sophisticated navigation system enables bacteria to thrive in complex and changing environments.
Pili (Fimbriae): The Adhesion Specialists
While flagella are primarily responsible for movement, pili (or fimbriae) are hair-like appendages that enable bacteria to adhere to surfaces, host cells, and other bacteria. These structures are typically shorter and thinner than flagella and are present in large numbers on the cell surface.
Structure and Function of Pili
Pili are composed of protein subunits called pilins. They extend from the cell surface and can bind to specific receptors on other cells or surfaces. This binding is highly specific, allowing bacteria to colonize particular environments or infect specific hosts.
Role in Biofilm Formation
Pili play a crucial role in the formation of biofilms. Biofilms are complex communities of bacteria embedded in a self-produced matrix of extracellular polymeric substances (EPS). These communities are highly resistant to antibiotics and other antimicrobial agents, making them a significant challenge in clinical settings. Pili enable bacteria to initially attach to a surface, a critical first step in biofilm formation. Once attached, the bacteria can then multiply and produce EPS, leading to the development of a mature biofilm.
Internal Components: The Machinery of Life
Having examined the external defenses and appendages that bacteria utilize, it is time to venture inside the cell and explore the inner workings that sustain life at this microscopic scale. The bacterial cell, though lacking the complex organelles of eukaryotes, possesses a meticulously organized internal environment. This environment allows it to perform all the essential functions of life. Within this microscopic realm lie the cytoplasm, ribosomes, nucleoid, and plasmids – each playing a critical role in the cell’s survival and replication.
Cytoplasm: The Foundation of Cellular Activity
The cytoplasm is the gel-like substance that fills the interior of the bacterial cell. This aqueous environment is far from being a simple void; rather, it is a complex mixture of water, enzymes, nutrients, and genetic material. These components are essential for the myriad of biochemical reactions that keep the cell alive.
It serves as the site for crucial processes such as metabolism, protein synthesis, and DNA replication. The cytoplasm provides a medium for the transport of molecules and the interaction of cellular components.
Without the cytoplasm, the bacterial cell would lack the structural and chemical foundation necessary to support life processes.
Ribosomes: Protein Synthesis Centers
Protein synthesis, the creation of proteins from genetic instructions, is a cornerstone of life. In bacteria, this vital process is carried out by ribosomes. Bacterial ribosomes, known as 70S ribosomes, are smaller than their eukaryotic counterparts (80S ribosomes). This difference is significant and is often exploited by antibiotics that target bacterial ribosomes without harming human cells.
These ribosomes are composed of two subunits: a 30S subunit and a 50S subunit. Each subunit contains ribosomal RNA (rRNA) and ribosomal proteins.
During protein synthesis, ribosomes bind to messenger RNA (mRNA) and transfer RNA (tRNA). tRNA molecules deliver amino acids to the ribosome, where they are linked together to form a polypeptide chain. This chain then folds into a functional protein. The efficiency and accuracy of ribosomes are critical for bacterial survival, as they ensure the production of the proteins necessary for all cellular functions.
Nucleoid (DNA): The Genetic Command Center
Unlike eukaryotic cells, bacteria lack a membrane-bound nucleus. Instead, their genetic material is concentrated in a region of the cytoplasm called the nucleoid. The bacterial nucleoid consists of a single, circular chromosome composed of DNA.
This chromosome contains all the genetic information required for the cell's survival and reproduction. The DNA within the nucleoid is highly organized and compacted. It is achieved through supercoiling and association with proteins.
This ensures that the lengthy DNA molecule fits within the confines of the bacterial cell. The nucleoid is the control center of the bacterial cell. It directs all cellular activities through the processes of DNA replication, transcription, and translation.
Plasmids: Extrachromosomal Elements
In addition to the main chromosome, many bacteria also contain plasmids. These are small, circular DNA molecules that exist independently of the chromosome. Plasmids are not essential for bacterial survival under normal conditions. However, they often carry genes that provide a selective advantage.
These genes can include antibiotic resistance genes and virulence factors. Antibiotic resistance genes enable bacteria to survive exposure to antibiotics. This is a major concern in healthcare settings.
Virulence factors enhance the ability of bacteria to cause disease. Plasmids can be transferred between bacteria through a process called conjugation. This facilitates the rapid spread of antibiotic resistance and virulence. The presence of plasmids can significantly impact bacterial adaptability and pathogenicity.
Survival Structures: Endospores - The Dormant Warriors
Life at the microbial level is a constant struggle against fluctuating environmental conditions. Bacteria, masters of adaptation, have evolved a remarkable survival strategy to endure extreme stress. Among the most resilient of these adaptations is the formation of endospores, dormant structures that allow certain bacteria to withstand conditions that would otherwise be lethal. These "dormant warriors" are not reproductive structures, but rather a survival mechanism, ensuring the preservation of the bacterial genome during times of adversity.
The Genesis of an Endospore: Sporulation
The process of endospore formation, known as sporulation, is triggered by environmental stress. Typically, nutrient deprivation is the primary instigator. When a bacterium senses a lack of essential nutrients, it initiates a complex cascade of gene expression. This cascade ultimately leads to the creation of a highly resistant, metabolically inactive endospore within the vegetative cell.
The process is remarkably ordered:
- The bacterial DNA is duplicated and isolated.
- The cell membrane invaginates to form a forespore.
- A second membrane layer engulfs the forespore, creating a double-layered structure.
- The peptidoglycan cortex is formed between these two layers.
- A tough protein coat is assembled around the cortex.
- Finally, the mother cell lyses, releasing the mature endospore.
Endospore Structure: A Fortress Within a Cell
The endospore's extraordinary resistance stems from its unique and complex structure.
-
The Core: At the heart of the endospore lies the core. This contains the bacterial DNA, ribosomes, and essential enzymes, all in a dehydrated state.
-
The Inner Membrane: Surrounding the core is the inner membrane. This acts as a permeability barrier.
-
The Cortex: The cortex is a thick layer of peptidoglycan. It is less cross-linked than that found in the vegetative cell wall.
-
The Spore Coat: This is a tough, proteinaceous layer. It provides protection from chemicals, enzymes, and physical damage.
-
The Exosporium: In some species, an outermost, loose-fitting covering known as the exosporium further protects the endospore.
Resistance to Extremes: The Indomitable Spore
Endospores are renowned for their ability to withstand extreme environmental conditions. This includes heat, radiation, desiccation, chemical disinfectants, and even starvation. This remarkable resistance is attributed to several factors:
-
Dehydration: The low water content within the endospore core increases resistance to heat and radiation.
-
Dipicolinic Acid (DPA): This is a unique chemical found in high concentrations within endospores. It stabilizes DNA and helps to dehydrate the core.
-
Small Acid-Soluble Proteins (SASPs): These proteins bind to DNA and protect it from damage. They also serve as a carbon and energy source during germination.
-
Impermeability: The spore coat and inner membrane act as barriers. They prevent the entry of harmful chemicals.
From Dormancy to Activity: Germination and Outgrowth
When environmental conditions become favorable, endospores can revert to their metabolically active, vegetative state. This process involves three distinct stages:
-
Activation: This often requires a trigger, such as heat or nutrient exposure, to prepare the spore for germination.
-
Germination: This is a rapid process. It involves the uptake of water, loss of DPA, degradation of the cortex, and increased metabolic activity.
-
Outgrowth: This is the final stage. The vegetative cell emerges from the spore coat and begins to grow and divide.
Endospores and Disease: A Threat to Human Health
While endospores are essential for bacterial survival, their resilience also poses a threat to human health. Several pathogenic bacteria, including Bacillus anthracis (anthrax) and Clostridium species (tetanus, botulism, gas gangrene, and Clostridioides difficile infection), form endospores. These spores can persist in the environment for extended periods and cause infection when they enter the body.
Effective sterilization techniques, such as autoclaving, are crucial for eliminating endospores from medical equipment and food products. Understanding the mechanisms of endospore formation, resistance, and germination is essential for developing new strategies to combat these resilient pathogens and prevent disease.
Microscopy Techniques: Peering into the Bacterial World
Having explored the intricate architecture of bacterial cells, a natural question arises: how do scientists observe these microscopic marvels? The answer lies in the powerful realm of microscopy, a collection of techniques that allows us to visualize structures far beyond the reach of the naked eye. Microscopy is essential for unlocking the secrets of bacterial morphology, arrangement, and internal organization, providing crucial insights into their behavior, function, and interactions with their environment.
Light Microscopy: A Window into Living Bacteria
Light microscopy, the workhorse of microbiology, utilizes visible light to illuminate and magnify specimens. This technique is relatively simple, inexpensive, and allows for the observation of living bacteria, making it invaluable for studying bacterial motility, division, and response to stimuli in real-time.
Bright-Field Microscopy
Bright-field microscopy, the most basic form of light microscopy, illuminates the sample with white light, creating a bright background against which stained or naturally pigmented bacteria can be observed. While useful for visualizing bacterial shape and arrangement, bright-field microscopy provides limited contrast for unstained, transparent bacteria.
Phase Contrast Microscopy
Phase contrast microscopy enhances the contrast of transparent specimens by exploiting differences in the refractive index of cellular components. This technique allows for the visualization of unstained bacteria, revealing internal structures and improving the overall image quality compared to bright-field microscopy.
Fluorescence Microscopy
Fluorescence microscopy employs fluorescent dyes or proteins to label specific bacterial structures or molecules. When illuminated with specific wavelengths of light, these fluorescent markers emit light of a different wavelength, allowing for the visualization of targeted components within the cell. Fluorescence microscopy is crucial for studying protein localization, gene expression, and bacterial interactions with host cells.
Electron Microscopy: Revealing the Nanoscale World
Electron microscopy utilizes beams of electrons instead of light to image specimens, achieving much higher magnifications and resolutions than light microscopy. This allows for the visualization of bacterial structures at the nanoscale, revealing intricate details of cell walls, ribosomes, and internal membranes. However, electron microscopy typically requires extensive sample preparation, including fixation and staining, which can alter or damage the specimen. Also, electron microscopy cannot be used to view living specimens.
Transmission Electron Microscopy (TEM)
TEM directs a beam of electrons through a thin section of the specimen, creating a two-dimensional image based on the electron density of different cellular components. TEM is ideal for visualizing the internal structures of bacteria, such as the nucleoid, ribosomes, and membranes.
Scanning Electron Microscopy (SEM)
SEM scans the surface of the specimen with a focused beam of electrons, creating a three-dimensional image based on the electrons that are scattered or emitted from the surface. SEM provides detailed information about the surface topography of bacteria, including the cell wall, flagella, and pili.
Choosing the Right Tool for the Task
The choice of microscopy technique depends on the specific research question and the nature of the sample. Light microscopy is well-suited for observing living bacteria and studying dynamic processes, while electron microscopy is necessary for visualizing nanoscale structures and obtaining high-resolution images. By combining different microscopy techniques, scientists can gain a comprehensive understanding of bacterial structure and function, paving the way for new discoveries in microbiology and related fields.
Video: Bacterial Structure: The Ultimate Guide You NEED to Know
Frequently Asked Questions About Bacterial Structure
This section addresses common questions about bacterial structure, providing quick and clear answers to help you better understand these fascinating microorganisms.
What are the main differences between Gram-positive and Gram-negative bacterial structure?
The key difference lies in the cell wall. Gram-positive bacteria have a thick peptidoglycan layer as their primary cell wall component. Gram-negative bacteria have a thin peptidoglycan layer and an outer membrane containing lipopolysaccharide (LPS). This outer membrane is a key structural feature.
What is the purpose of the bacterial capsule?
The capsule is a protective outer layer found in some bacteria. Its primary functions include aiding in adherence to surfaces, resisting phagocytosis by immune cells, and preventing desiccation. Thus, it directly impacts bacterial structure and survival.
How do bacterial flagella contribute to bacterial structure and function?
Flagella are whip-like appendages used for bacterial motility. Their structure consists of a filament, hook, and basal body. They allow bacteria to move towards nutrients or away from harmful substances, playing a crucial role in their survival and spread.
What is the significance of the bacterial cell membrane?
The cell membrane, also known as the plasma membrane, encloses the bacterial cytoplasm and is essential for its function. It regulates the passage of substances in and out of the cell, and is also involved in energy production and cell wall synthesis. Therefore, it's a vital component of bacterial structure.