ATP & ADP: The Energy Secrets Your Body Hides! #Biohack
Understanding the intricate dance of ATP and ADP molecule is fundamental to unlocking the energy secrets within our bodies. Mitochondria, the cellular powerhouses, are the primary sites for ATP synthesis. This process, often studied within the framework of biohacking, involves ADP accepting a phosphate group, thus forming the energy-rich ATP molecule. The energy released from ATP's hydrolysis then powers diverse cellular functions, including muscle contraction and nerve impulse transmission. Ultimately, mastering the atp and adp molecule is key to understanding human performance and longevity.
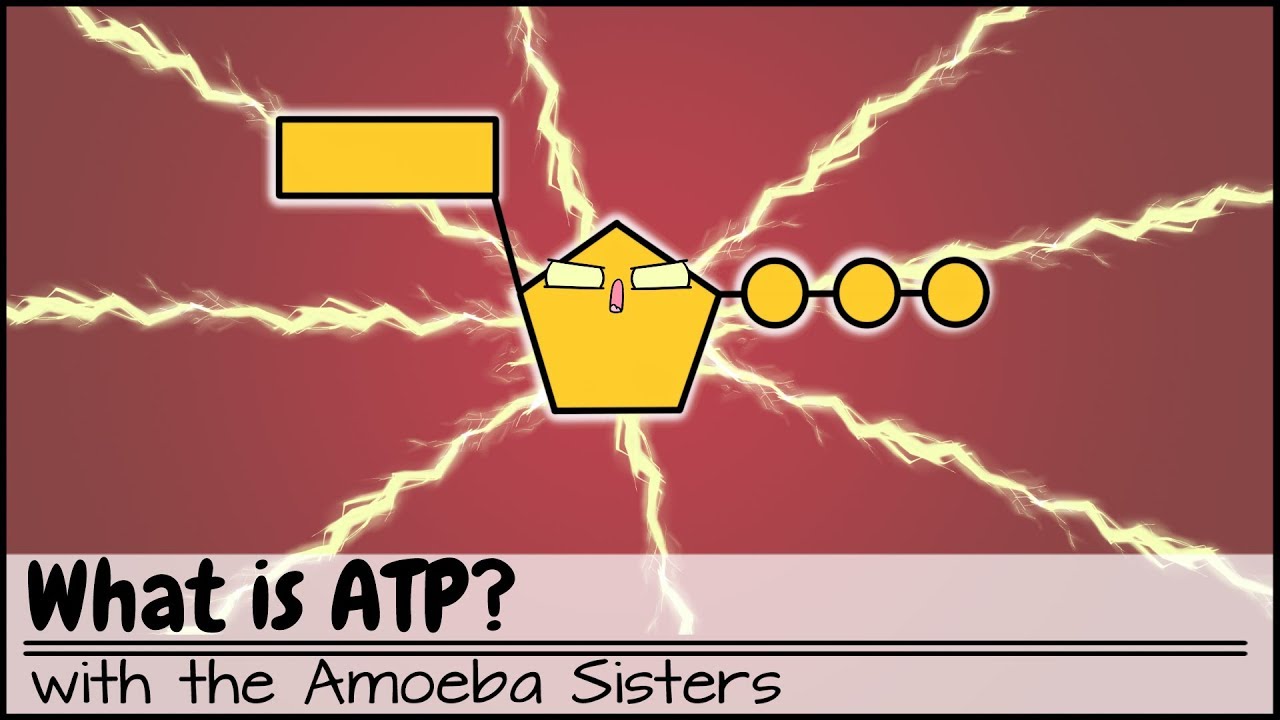
Image taken from the YouTube channel Amoeba Sisters , from the video titled What is ATP? .
Unlocking the Energy Secrets Within
Imagine a microscopic world, a constant flurry of activity, where every movement, thought, and breath is powered by an unseen engine. This engine, the very essence of life, operates on a molecular currency known as ATP and ADP. These molecules are not just components of our cells; they are the fundamental units of energy that drive our existence.
The Unseen Engine of Life
Every second, trillions of ATP (adenosine triphosphate) molecules are synthesized and broken down within our bodies. This constant cycle fuels everything from muscle contraction to nerve impulse transmission, from DNA replication to protein synthesis. It is a dynamic and intricate process, and understanding it is key to unlocking the secrets of our own energy potential.
ATP and ADP: The Energy Currency Explained
ATP, often called the "energy currency" of the cell, is a complex molecule that stores energy in its chemical bonds. When a cell needs energy, ATP is broken down into ADP (adenosine diphosphate) and a phosphate group. This process, known as hydrolysis, releases energy that the cell can use to perform work.
Conversely, ADP can be converted back into ATP through a process called phosphorylation, where a phosphate group is added back to the ADP molecule. This recharging process requires energy, which is derived from the breakdown of food molecules through cellular respiration. This constant cycle of ATP breakdown and regeneration ensures a continuous supply of energy to power cellular functions.
Optimizing Energy: The Key to Health and Performance
Understanding the roles of ATP and ADP is not just an academic exercise. It has profound implications for our overall health, well-being, and performance. By optimizing our ATP production, we can increase our energy levels, improve our physical and mental performance, and even slow down the aging process.
This exploration into the world of ATP and ADP will equip you with the knowledge to harness the power of these energy molecules. You'll be empowered to make informed choices about your diet, exercise, and lifestyle. Through those choices, you can optimize your energy levels and unlock your full potential.
Optimizing Energy: The Key to Health and Performance requires understanding the intricate dance of energy within our cells. The key players in this performance are ATP and ADP. But before diving into the nuances of optimizing their function, it's crucial to establish a clear understanding of what these molecules are and how they relate to each other.
ATP and ADP: The Dynamic Duo Defined
At the heart of cellular energy transfer lie two molecules, ATP (adenosine triphosphate) and ADP (adenosine diphosphate). These molecules are not just chemical compounds; they are the fundamental units that power nearly every biological process. Understanding their structure, relationship, and roles is essential for grasping how energy is stored and utilized within living organisms.
Defining ATP (Adenosine Triphosphate)
ATP, or adenosine triphosphate, is often referred to as the primary energy currency of the cell. Its structure comprises three key components:
- Adenine: A nitrogenous base.
- Ribose: A five-carbon sugar.
- Triphosphate Group: A chain of three phosphate groups.
It is within the bonds of these phosphate groups that energy is stored. The bonds that hold the phosphate groups together are high-energy bonds. When one of these bonds is broken through hydrolysis, energy is released that the cell can then use to perform work.
ATP's function is not merely to store energy, but to readily release it when and where it's needed. This makes it the ideal energy carrier for the diverse and dynamic processes within a cell.
Defining ADP (Adenosine Diphosphate)
ADP, or adenosine diphosphate, is closely related to ATP. It is essentially ATP that has lost one of its phosphate groups.
- It also consists of adenine and ribose.
- The difference is it only has two phosphate groups attached.
ADP is often considered the lower-energy form of ATP.
When ATP is used to power cellular processes, it is broken down into ADP and a phosphate group. The energy released fuels the necessary reactions. ADP is then available to be "recharged" back into ATP, thus continuing the energy cycle.
This relationship between ATP and ADP is central to energy transfer within cells.
The ATP/ADP Cycle: Charge and Recharge
The constant interconversion of ATP and ADP forms a cyclical process that drives cellular activity.
Here's how the cycle works:
-
ATP Hydrolysis: When a cell requires energy, ATP is hydrolyzed (broken down using water). This cleaves off one phosphate group. This generates ADP, a phosphate group, and energy.
-
Energy Utilization: The energy released from ATP hydrolysis is used to power various cellular functions, such as muscle contraction, nerve impulse transmission, and protein synthesis.
-
ADP Phosphorylation: To replenish the energy supply, ADP undergoes phosphorylation. Phosphorylation is a process where a phosphate group is added back to the ADP molecule. This converts ADP back into ATP.
-
Energy Input: This "recharging" process requires an input of energy. This energy is derived from the breakdown of food molecules, primarily through cellular respiration.
This continuous cycle of ATP breakdown and regeneration ensures a constant supply of energy to power cellular functions. It's a dynamic equilibrium that allows cells to respond quickly to changing energy demands.
ATP and ADP, as we've established, are the immediate energy currencies of the cell. But why is this energy so essential in the first place? The answer lies in the fact that energy is the driving force behind every single biological process, from the simplest cellular activity to the most complex functions of a multicellular organism.
The Crucial Role of Energy in Biological Processes
Without a constant supply of energy, life as we know it would cease to exist. ATP and ADP are not merely players in a metabolic equation; they are the lifeblood of our cells, fueling existence itself.
The Indispensable Nature of Energy
Energy is not just a luxury for living organisms; it is an absolute necessity. It powers the myriad of processes that keep us alive and functioning.
Maintaining homeostasis, the delicate balance of internal conditions, is an energy-intensive task. From regulating body temperature to maintaining proper pH levels, our bodies are constantly expending energy to stay within optimal ranges.
Growth, repair, and reproduction – all fundamental aspects of life – demand significant energy input. Every cell division, every protein synthesized, and every tissue repaired requires a continuous stream of ATP.
Movement, whether it's a single-celled organism propelling itself or a human running a marathon, is perhaps the most obvious example of energy expenditure.
Cellular Respiration: The Energy Extraction Process
Cellular respiration is the metabolic pathway by which cells extract energy from nutrients, primarily glucose. It's a complex process that involves a series of chemical reactions, ultimately converting the energy stored in food into a usable form: ATP.
This process can be summarized as follows: glucose + oxygen → carbon dioxide + water + energy (ATP).
ATP is produced during several stages of cellular respiration, most notably during oxidative phosphorylation in the mitochondria. Thus, cellular respiration directly replenishes the ATP pool, ensuring a constant supply of energy for cellular functions.
This continuous cycle of energy extraction, conversion to ATP, and utilization is the very essence of life.
ATP and ADP Functions: Beyond the Basics
ATP and ADP's roles extend far beyond simply providing energy for general cellular functions. They are intimately involved in highly specific and critical bodily processes.
Muscle Contraction: Powering Movement
Muscle contraction, the very act of movement, is driven by ATP. The protein myosin uses ATP to bind to actin filaments and pull them, causing muscle fibers to shorten. This process requires a continuous supply of ATP to sustain muscle activity.
When ATP is depleted, muscles become fatigued and unable to contract, leading to muscle cramps and exhaustion.
Nerve Impulses: Sending Signals
Nerve impulse transmission, the communication network of our nervous system, also depends on ATP. Neurons use ATP to maintain the ion gradients across their cell membranes, which are essential for generating and transmitting electrical signals.
The sodium-potassium pump, a key protein in nerve cells, uses ATP to actively transport ions across the membrane, creating the electrochemical gradient necessary for nerve impulse propagation. This ensures rapid and accurate signal transmission throughout the body.
Disruptions in ATP supply can impair nerve function, leading to neurological disorders and impaired cognitive function. These are just two examples that show that ATP and ADP are indispensable for a multitude of biological functions.
The indispensable nature of energy highlights the importance of understanding where this life-sustaining force originates. While ATP and ADP provide the immediate energy currency, the cellular structures responsible for generating this currency are equally critical.
Mitochondria: The ATP Powerhouse
Within the intricate landscape of the cell, a specialized organelle reigns supreme as the primary site of ATP production: the mitochondrion. Often hailed as the "powerhouse of the cell," mitochondria are the energy factories that fuel nearly all eukaryotic life. Their importance in cellular energy metabolism cannot be overstated, as they are the linchpin connecting nutrient breakdown to the generation of usable energy.
Unveiling the Powerhouse
Mitochondria are complex organelles characterized by their distinctive double-membrane structure. The outer membrane is smooth, while the inner membrane is highly folded into structures called cristae. These cristae increase the surface area available for the crucial chemical reactions that generate ATP.
The space between the two membranes is the intermembrane space, and the space enclosed by the inner membrane is the mitochondrial matrix. This intricate architecture is not merely for show; it plays a vital role in the efficiency of ATP production.
The Cellular Respiration Connection
Mitochondria are inextricably linked to cellular respiration, the metabolic process that extracts energy from glucose and other nutrients. While the initial stages of cellular respiration, such as glycolysis, occur in the cytoplasm, the majority of ATP is generated within the mitochondria.
The Krebs cycle (also known as the citric acid cycle) and the electron transport chain – two key stages of cellular respiration – take place within the mitochondrial matrix and inner membrane, respectively. These processes harness the energy stored in nutrient molecules to create a proton gradient, which drives the synthesis of ATP.
ATP Production Significance
The significance of mitochondria in ATP production stems from their unique ability to carry out oxidative phosphorylation. This process, which occurs along the electron transport chain, uses the energy from electrons to pump protons across the inner mitochondrial membrane, creating an electrochemical gradient.
This gradient then drives ATP synthase, a remarkable enzyme complex that acts as a molecular turbine, converting the energy of the proton gradient into the chemical energy of ATP. It's estimated that mitochondria produce over 90% of the ATP required by most cells, underscoring their critical role in fueling cellular activities. Without functional mitochondria, cells would rapidly run out of energy, leading to cellular dysfunction and ultimately, cell death.
Mitochondria are inextricably linked to cellular respiration, the metabolic process that extracts energy from glucose and other nutrients. While the initial stages of cellular respiration, such as glycolysis, occur in the cytoplasm, the majority of ATP is generated within the mitochondria.
The Krebs cycle (also known as the citric acid cycle) and the electron transport chain – two key processes in cellular respiration – both take place within this organelle. But to fully grasp the energy-generating prowess of mitochondria, it is essential to understand the continuous cycle that underpins their function: the ATP/ADP cycle.
The ATP/ADP Cycle: A Detailed Look
The ATP/ADP cycle is the fundamental process by which cells manage energy. It is a continuous loop of energy expenditure and replenishment, involving the breakdown of ATP (adenosine triphosphate) to release energy and the subsequent regeneration of ATP from ADP (adenosine diphosphate).
This cycle is crucial for sustaining all energy-dependent biological functions. Understanding the intricacies of this cycle provides profound insights into how cells efficiently harness and distribute energy.
Hydrolysis: Breaking Down ATP
Hydrolysis is the chemical process at the heart of ATP's energy-releasing capabilities. It involves the breaking of a phosphate bond in ATP through the addition of a water molecule (H₂O).
This process converts ATP into ADP, releasing a phosphate group (Pi) and a significant amount of energy that the cell can then use to perform work.
The Chemistry of Hydrolysis
At a molecular level, hydrolysis involves the nucleophilic attack of a water molecule on one of the phosphate groups of ATP. This breaks the bond connecting that phosphate group to the rest of the ATP molecule.
The reaction can be represented as: ATP + H₂O → ADP + Pi + Energy
The released phosphate group is not just a byproduct; it often plays a regulatory role in various cellular processes.
Energy Release During Hydrolysis
The energy released during ATP hydrolysis is substantial, typically around 7.3 kilocalories per mole (kcal/mol) under standard conditions. This energy can be harnessed to power a wide array of cellular activities, including:
- Muscle contraction
- Active transport of molecules across cell membranes
- Synthesis of proteins and other biomolecules
- Nerve impulse transmission
The amount of energy released is highly dependent on cellular conditions, such as pH and ion concentrations, which fine-tune the process to meet specific energy demands.
Phosphorylation: Recharging ADP
While hydrolysis extracts energy from ATP, phosphorylation is the process that replenishes it. Phosphorylation involves the addition of a phosphate group (Pi) to ADP to reform ATP. This "recharging" process requires energy input, effectively storing energy within the newly formed phosphate bond.
The Process of Phosphorylation
Phosphorylation is not a spontaneous reaction; it requires energy input to overcome the energetic barrier. This energy is derived from various sources depending on the type of phosphorylation.
For instance, in cellular respiration, the energy released from the breakdown of glucose is used to drive phosphorylation. The reaction can be represented as: ADP + Pi + Energy → ATP
The newly synthesized ATP molecule is then ready to undergo hydrolysis, restarting the energy cycle.
Types of Phosphorylation
Several different mechanisms facilitate phosphorylation, each playing a unique role in cellular energy metabolism. Key types include:
-
Substrate-Level Phosphorylation: This direct transfer of a phosphate group from a high-energy intermediate molecule to ADP occurs during glycolysis and the Krebs cycle. It generates a small amount of ATP.
-
Oxidative Phosphorylation: This is the primary mechanism for ATP production in aerobic organisms. It occurs in the mitochondria during the electron transport chain. The energy released from the transfer of electrons is used to pump protons (H+) across the inner mitochondrial membrane, creating an electrochemical gradient. This gradient then drives ATP synthase, an enzyme complex that phosphorylates ADP to produce ATP.
- Oxidative phosphorylation is significantly more efficient than substrate-level phosphorylation. It generates the vast majority of ATP required by the cell.
Understanding the ATP/ADP cycle, with its intricate processes of hydrolysis and phosphorylation, provides a window into the fundamental mechanisms that power life. This cycle is not merely a biochemical pathway. It is the energetic foundation upon which all cellular processes are built, making it essential for comprehending the dynamics of biological energy.
Enzymes: The Catalysts of ATP/ADP Conversion
Having explored the dynamic ATP/ADP cycle, it's essential to recognize the unsung heroes orchestrating this energy dance: enzymes. These biological catalysts significantly accelerate the interconversion between ATP and ADP, ensuring that energy is available precisely when and where it's needed within the cell. Without enzymes, the energy transactions within our bodies would be far too slow to sustain life.
Key Enzymes Involved in ATP/ADP Conversion
Enzymes don't just facilitate reactions; they are intricately woven into the very fabric of metabolic processes. Two enzyme families stand out in the ATP/ADP story: ATP synthase and kinases.
ATP Synthase: The Molecular Turbine
ATP synthase is perhaps the most remarkable enzyme in the entire process. Functioning like a miniature molecular turbine, ATP synthase harnesses the energy of a proton gradient across the inner mitochondrial membrane to synthesize ATP.
This gradient, established by the electron transport chain, drives protons (H+) through ATP synthase. The flow of protons causes a rotor within the enzyme to spin, mechanically driving the phosphorylation of ADP to form ATP.
It’s an exquisite example of chemiosmosis, where chemical energy is generated through a membrane. The process is highly efficient, converting potential energy stored in the proton gradient into the readily usable chemical energy of ATP.
Kinases: Phosphate Transfer Masters
Kinases represent a broad family of enzymes with a diverse range of functions, all centered on one key action: transferring phosphate groups from ATP to other molecules. This process, known as phosphorylation, is a fundamental regulatory mechanism in cells.
When a kinase transfers a phosphate group to a target protein, it can alter that protein's activity, conformation, or interaction with other molecules. Think of it as a biological on/off switch.
Kinases control numerous cellular processes, including signal transduction, metabolism, and cell cycle regulation. The importance of kinases in disease is highlighted by the fact that many cancer therapies target specific kinases involved in uncontrolled cell growth.
The Importance of Enzymes as Biological Catalysts
Enzymes are not merely passive participants in the ATP/ADP cycle. They are active drivers that dictate the rate and efficiency of energy transfer. Without these biological catalysts, the reactions involved in ATP hydrolysis and phosphorylation would occur far too slowly to sustain life.
Enzymes provide a specific environment within their active sites that lowers the activation energy required for a reaction to occur. They orient substrates correctly, stabilize transition states, and sometimes even directly participate in the chemical reaction.
Enzymes are also highly regulated, responding to various cellular signals to fine-tune their activity. This regulation ensures that ATP production and utilization are precisely matched to the cell's energy demands, maintaining a delicate balance crucial for cellular health and survival.
ATP/ADP and Metabolic Pathways: A Complex Interplay
The story of ATP and ADP isn't confined to single enzymatic reactions; it's a sprawling narrative woven into the very fabric of metabolic pathways. These pathways, intricate sequences of chemical reactions, dictate how our bodies extract energy from food and channel it into vital functions. ATP and ADP act as both key players and crucial regulators within these metabolic orchestrations.
ATP and ADP: Metabolic Regulators
ATP and ADP exert powerful control over metabolic pathways, acting as signals that either accelerate or decelerate specific reactions. This regulation often operates through allosteric modulation, where ATP or ADP binds to an enzyme at a site distinct from its active site, subtly altering the enzyme's shape and activity.
A high ATP concentration often indicates an energy-rich state, signaling a slowdown in ATP-generating pathways. Conversely, elevated ADP levels typically reflect an energy deficit, prompting the activation of processes that boost ATP production.
This feedback mechanism ensures a delicate balance, preventing wasteful overproduction or dangerous energy depletion.
Glycolysis: Unlocking Glucose's Potential
Glycolysis, the breakdown of glucose into pyruvate, stands as the initial stage of cellular respiration. This pathway generates a small amount of ATP directly, but its primary role is to prepare glucose-derived molecules for further energy extraction.
The enzymes within glycolysis are highly sensitive to ATP and ADP levels. For example, phosphofructokinase (PFK), a key regulatory enzyme, is inhibited by high ATP concentrations, preventing excessive glucose breakdown when energy is plentiful. ADP, on the other hand, activates PFK, stimulating glycolysis when energy is scarce. This fine-tuned control ensures that glucose is utilized efficiently, meeting the cell's energy demands without wasteful overconsumption.
Krebs Cycle (Citric Acid Cycle): The Metabolic Hub
The Krebs cycle, also known as the citric acid cycle, is a central metabolic hub, oxidizing acetyl-CoA (derived from pyruvate and fatty acids) to generate high-energy electron carriers (NADH and FADH2) and a small amount of ATP. While ATP's direct regulatory role within the Krebs cycle isn't as pronounced as in glycolysis, the cycle's overall activity is indirectly influenced by the cellular ATP/ADP ratio.
A high ATP/ADP ratio suggests that the electron transport chain is operating effectively, maintaining a sufficient supply of NAD+ and FAD+, essential for the Krebs cycle to function optimally. Conversely, a low ATP/ADP ratio may indicate a bottleneck in the electron transport chain, leading to an accumulation of NADH and FADH2, which can slow down the Krebs cycle.
Electron Transport Chain: The Ultimate ATP Generator
The electron transport chain (ETC) is the final stage of cellular respiration, where the high-energy electrons carried by NADH and FADH2 are used to pump protons across the inner mitochondrial membrane, creating an electrochemical gradient. This gradient then drives ATP synthase, the molecular turbine that directly produces the vast majority of cellular ATP.
The ETC is tightly coupled to oxidative phosphorylation; the flow of electrons is directly linked to ATP synthesis. ADP availability is a critical factor regulating the rate of electron transport. When ADP levels are high, signaling a need for more ATP, the ETC accelerates to replenish the ATP supply. Conversely, when ATP levels are high and ADP levels are low, the ETC slows down, preventing excessive ATP production and minimizing the generation of reactive oxygen species (ROS).
The interplay between ATP, ADP, and the electron transport chain ensures that energy production is precisely matched to cellular demand.
ATP and ADP exert powerful control over metabolic pathways, acting as signals that either accelerate or decelerate specific reactions. This intricate regulation ensures a delicate balance, preventing wasteful overproduction or dangerous energy depletion. Now, let's delve into some specific examples of how ATP and ADP drive crucial bodily functions, illustrating their real-world impact on our daily lives.
ATP and ADP in Action: Powering Body Functions
ATP and ADP are not just theoretical molecules floating within our cells; they are the driving force behind countless physiological processes that enable us to move, think, and even breathe. From the simple act of lifting a finger to the complex transmission of nerve signals, these energy currencies are indispensable.
Muscle Contraction: Powering Movement
Muscle contraction, the very essence of movement, relies heavily on ATP. Myosin, a motor protein within muscle fibers, binds to actin filaments, forming cross-bridges. It's the hydrolysis of ATP that provides the energy for myosin to pull on actin, shortening the muscle fiber and generating force.
ADP is then released, and a new ATP molecule binds, allowing the cycle to repeat. Without a constant supply of ATP, muscles would be unable to contract and relax properly, leading to stiffness and fatigue.
The Sliding Filament Theory
The mechanics of muscle contraction is described by the sliding filament theory. This theory highlights how actin and myosin filaments interact. ATP fuels the cyclical attachment, pulling, and detachment of these filaments, resulting in muscle shortening.
Rigor Mortis: A Stark Reminder
Rigor mortis, the stiffening of muscles after death, serves as a stark reminder of ATP's crucial role. Once ATP production ceases, myosin remains bound to actin, leading to a rigid state. This phenomenon underscores the absolute dependence of muscle function on a continuous ATP supply.
Nerve Impulses: Sending Signals Throughout the Body
Beyond muscle contraction, ATP and ADP play a vital role in nerve impulse transmission. Neurons, the specialized cells that transmit information throughout the nervous system, maintain electrochemical gradients across their cell membranes.
These gradients are essential for generating and propagating electrical signals called action potentials. The sodium-potassium pump, an enzyme that actively transports ions across the neuronal membrane, requires ATP to maintain these gradients.
The Sodium-Potassium Pump
This pump, crucial for neuronal function, uses the energy from ATP hydrolysis to move sodium ions out of the cell and potassium ions into the cell. This creates a charge difference across the membrane, which is vital for transmitting nerve impulses.
Synaptic Transmission
At synapses, the junctions between neurons, ATP is also involved in the release and reuptake of neurotransmitters, chemical messengers that transmit signals from one neuron to another. This process ensures the efficient and accurate transmission of information throughout the nervous system.
In essence, ATP and ADP are the unsung heroes of our bodies, tirelessly working to power every movement, thought, and sensation we experience. Understanding their importance underscores the need to support these energy systems through healthy lifestyle choices.
ATP and ADP exert powerful control over metabolic pathways, acting as signals that either accelerate or decelerate specific reactions. This intricate regulation ensures a delicate balance, preventing wasteful overproduction or dangerous energy depletion. Now, let's delve into some specific examples of how ATP and ADP drive crucial bodily functions, illustrating their real-world impact on our daily lives.
Factors Influencing ATP Production: Optimizing Your Energy
The human body's capacity to generate ATP is not a fixed trait but rather a dynamic process influenced by a myriad of lifestyle factors. Understanding these influences empowers us to optimize our energy production and unlock our full potential. Diet, exercise, stress management, and sleep all play crucial roles in dictating how efficiently our bodies create and utilize this vital energy currency. Let's explore these factors in detail.
Diet and Nutrition: Fueling Energy Production
The food we consume serves as the fundamental raw material for ATP synthesis. A balanced diet, rich in essential nutrients, provides the building blocks and cofactors necessary for the complex biochemical reactions that generate ATP.
Macronutrients: The Foundation of Energy
Carbohydrates, fats, and proteins are the primary macronutrients that fuel ATP production. Carbohydrates are broken down into glucose, which enters glycolysis, the Krebs cycle, and the electron transport chain. Fats are metabolized through beta-oxidation, feeding into the Krebs cycle. Proteins, while less efficient as an energy source, can be converted into glucose or Krebs cycle intermediates.
A diet deficient in any of these macronutrients can compromise ATP production. Restrictive diets or imbalances can lead to fatigue, reduced physical performance, and impaired cognitive function.
Micronutrients: Essential Catalysts
Vitamins and minerals, though required in smaller amounts, play vital roles as coenzymes and cofactors in ATP-generating pathways. B vitamins, such as niacin (B3), riboflavin (B2), and pantothenic acid (B5), are essential components of the electron transport chain.
Iron is crucial for hemoglobin, which carries oxygen needed for oxidative phosphorylation, and also for cytochromes, components of the electron transport chain. Magnesium is involved in numerous enzymatic reactions related to ATP metabolism. Deficiencies in these micronutrients can significantly impair ATP production.
To optimize energy production through diet, focus on:
- Consuming a balanced diet with adequate amounts of carbohydrates, healthy fats, and protein.
- Prioritizing whole, unprocessed foods rich in vitamins and minerals.
- Ensuring sufficient intake of B vitamins, iron, and magnesium through diet or supplementation if needed.
Exercise: Enhancing Mitochondrial Function and ATP Synthesis
Regular physical activity is a powerful stimulus for boosting ATP production. Exercise increases the demand for energy, prompting the body to adapt by enhancing mitochondrial function and increasing ATP synthesis capacity.
Mitochondrial Biogenesis
Endurance exercise, in particular, stimulates mitochondrial biogenesis, the process by which cells create new mitochondria. More mitochondria translate to greater ATP-producing capacity, leading to improved endurance and overall energy levels.
Enhanced Efficiency
Exercise also improves the efficiency of existing mitochondria. Regular training increases the expression of enzymes involved in oxidative phosphorylation, allowing mitochondria to generate more ATP per unit of oxygen consumed. This enhanced efficiency contributes to reduced fatigue and improved athletic performance.
Types of Exercise
Both aerobic and anaerobic exercise contribute to improved ATP production. Aerobic exercise enhances mitochondrial function and oxidative capacity, while anaerobic exercise increases the body's ability to generate ATP quickly through glycolysis. A combination of both types of exercise provides a comprehensive approach to optimizing energy production.
To harness the energy-boosting benefits of exercise:
- Engage in regular physical activity, including both aerobic and anaerobic exercises.
- Gradually increase the intensity and duration of workouts to stimulate mitochondrial adaptations.
- Ensure proper nutrition to support the increased energy demands of exercise.
Stress and Sleep: Impacting Metabolism and Energy Levels
Chronic stress and insufficient sleep can wreak havoc on ATP production, leading to fatigue, reduced cognitive function, and increased risk of chronic diseases. These factors disrupt hormonal balance and metabolic processes, ultimately impairing the body's ability to generate and utilize energy efficiently.
The Impact of Stress
Chronic stress triggers the release of stress hormones such as cortisol, which can negatively impact ATP production in several ways. Cortisol can impair mitochondrial function, reduce glucose uptake by cells, and promote inflammation, all of which hinder ATP synthesis. Additionally, chronic stress can deplete nutrient stores, further compromising energy production.
The Importance of Sleep
Sleep is essential for cellular repair and energy restoration. During sleep, the body replenishes glycogen stores, repairs damaged tissues, and consolidates memories. Sleep deprivation disrupts these processes, leading to reduced ATP production and impaired energy metabolism. Insufficient sleep also increases levels of stress hormones, further exacerbating the negative impact on energy levels.
To mitigate the negative effects of stress and sleep deprivation on ATP production:
- Practice stress-reducing techniques such as meditation, yoga, or deep breathing exercises.
- Prioritize sleep hygiene by establishing a regular sleep schedule, creating a relaxing bedtime routine, and optimizing the sleep environment.
- Ensure adequate nutrient intake to support adrenal function and energy metabolism during times of stress.
Video: ATP & ADP: The Energy Secrets Your Body Hides! #Biohack
FAQs: Unlocking ATP & ADP's Energy Secrets
Here are some common questions about ATP and ADP, the energy powerhouses of your body. Let's dive in and uncover how these molecules fuel everything you do!
What exactly are ATP and ADP?
ATP (adenosine triphosphate) and ADP (adenosine diphosphate) are crucial molecules for energy storage and transfer within cells. Think of ATP as the fully charged battery, and ADP as the partially discharged one. The atp and adp molecule are constantly cycling between these two states.
How does ATP actually provide energy to my body?
ATP releases energy when it loses a phosphate group, becoming ADP. This process, called hydrolysis, breaks the bond holding the phosphate, releasing energy that fuels various cellular processes like muscle contraction, nerve impulse transmission, and protein synthesis. Think of it as the atp and adp molecule doing work.
What happens to ADP after ATP releases energy?
After ATP converts to ADP, it gets "recharged" back into ATP. This recharge process requires energy from the food you eat through cellular respiration. Then atp and adp molecule is ready to provide more energy.
What's the connection between biohacking and optimizing ATP production?
Biohacking strategies often focus on maximizing mitochondrial function. Mitochondria are the powerhouses of the cell where ATP is generated. By improving mitochondrial health through diet, exercise, and supplements, you can enhance atp and adp molecule production, potentially boosting energy levels and overall performance.