Argon Electron Configuration: Properties & Reactivity
Argon, a noble gas recognized by the International Union of Pure and Applied Chemistry (IUPAC), exhibits a unique electron arrangement that dictates its chemical behavior. Specifically, the argon electron configuration, denoted as 1s²2s²2p⁶3s²3p⁶, signifies a completely filled outer electron shell, which contributes to its inherent stability. The stability, observed in applications within the semiconductor industry, reveals argon's inertness, resulting in minimal reactivity with other elements under normal conditions. Consequently, the octet rule in chemical bonding elucidates the reason argon's electron configuration results in its limited participation in forming chemical compounds, thereby influencing its widespread use as a protective atmosphere in welding and other industrial processes.
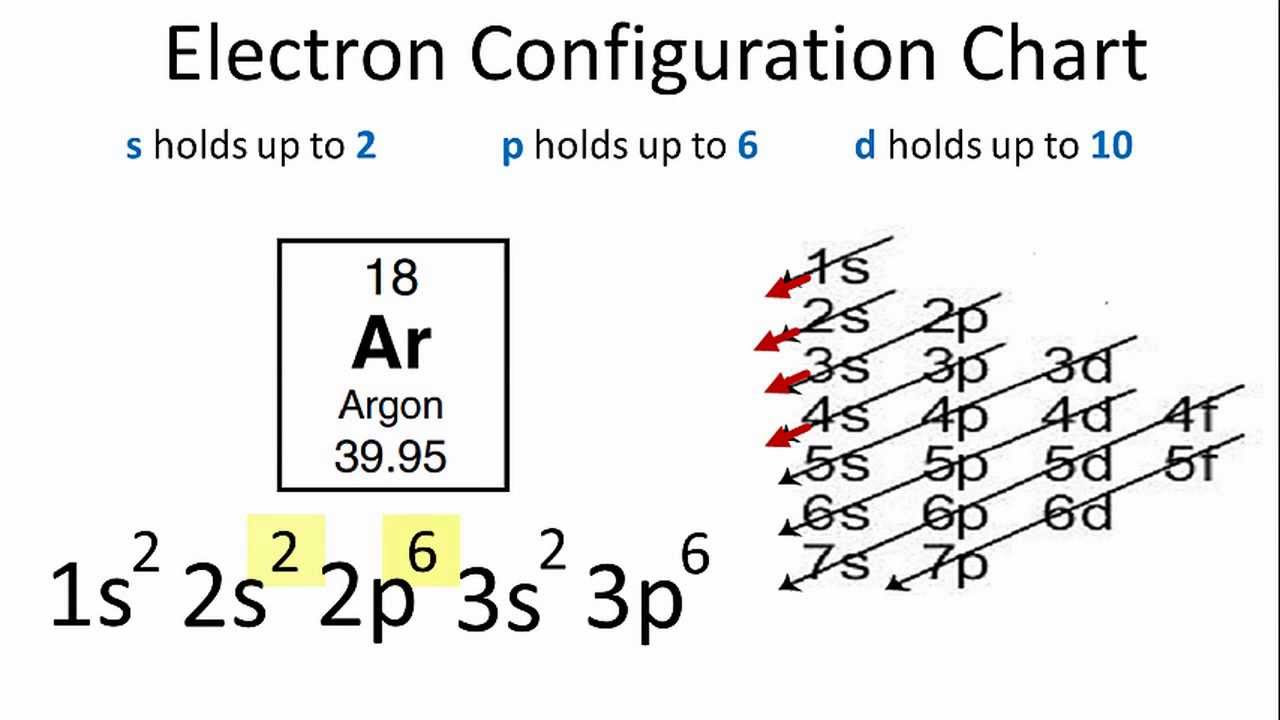
Image taken from the YouTube channel Wayne Breslyn (Dr. B.) , from the video titled Argon Electron Configuration .
Argon, symbolized as Ar and possessing the atomic number 18, stands as a quintessential noble gas. Its position in the periodic table, gracing Group 18, immediately signals its distinct chemical nature. This group is renowned for containing elements exhibiting remarkable inertness.
Significance of Electron Configuration
Understanding Argon's electron configuration is paramount to deciphering its characteristic lack of reactivity. The arrangement of its electrons dictates how it interacts, or rather, doesn't interact, with other elements.
Delving into this electronic structure reveals the underlying reasons for Argon's stability. This understanding extends beyond mere academic interest.
It provides a foundation for comprehending chemical bonding. It allows us to appreciate the behavior of other elements and compounds.
Blog Post Overview
This exploration will dissect Argon's electron configuration. We will elucidate the principles governing its arrangement.
We will address the octet rule and its impact on noble gas stability.
Furthermore, the discussion will extend to related properties. These include ionization energy and electronegativity.
Finally, we will reinforce the importance of electron configuration in understanding chemical behavior.
Atomic Number and Electron Arrangement: Setting the Stage
Argon, symbolized as Ar and possessing the atomic number 18, stands as a quintessential noble gas. Its position in the periodic table, gracing Group 18, immediately signals its distinct chemical nature. This group is renowned for containing elements exhibiting remarkable inertness.
Significance of Electron Configuration
Understanding Argon's electron configuration is paramount to deciphering the origins of this remarkable stability. It’s the key to unraveling the “why” behind Argon's reluctance to engage in chemical bonding under normal circumstances. Before diving into the specific electron distribution, let's establish the foundational principles that govern atomic structure.
Defining the Atomic Number: Argon's Identity
The atomic number, a cornerstone of atomic theory, defines an element's identity. It represents the number of protons residing within the nucleus of an atom.
For Argon, this number is 18. This dictates that every Argon atom possesses precisely 18 protons. In a neutral atom, the number of protons is balanced by an equal number of electrons, those negatively charged particles whirling around the nucleus. Consequently, a neutral Argon atom contains 18 electrons. This balance is fundamental to the atom's overall electrical neutrality.
Electron Shells: Energy Levels and Electron Capacity
Electrons aren't randomly scattered around the nucleus. Instead, they occupy specific energy levels, often referred to as electron shells. These shells are designated by the principal quantum number, n, taking integer values of 1, 2, 3, and so on. These correspond to the K, L, M shells and beyond, respectively.
Each shell can accommodate a limited number of electrons, dictated by the formula 2n². This determines the maximum electron capacity of each shell. The first shell (K, n=1) can hold up to 2 electrons. The second shell (L, n=2) can hold up to 8 electrons. The third shell (M, n=3) can hold up to 18 electrons, and so forth.
Atomic Orbitals: Refining Electron Location
While electron shells provide a general framework, a more precise description of electron location is achieved through the concept of atomic orbitals. Within each shell, electrons reside in specific regions of space known as atomic orbitals. These orbitals are characterized by distinct shapes and energy levels.
The four primary types of atomic orbitals are designated as s, p, d, and f. Each type can hold a specific number of electrons:
-
s orbitals: These are spherical and can hold a maximum of 2 electrons.
-
p orbitals: These are dumbbell-shaped and a set of three p orbitals can hold a maximum of 6 electrons.
-
d orbitals: These have more complex shapes and a set of five d orbitals can hold a maximum of 10 electrons.
-
f orbitals: These possess even more intricate shapes and a set of seven f orbitals can hold a maximum of 14 electrons.
Understanding these fundamental concepts of atomic number, electron shells, and atomic orbitals is critical to grasping the electron configuration of Argon. This understanding sets the stage for explaining its unique chemical properties.
Mapping the Electrons: Argon's Ground State Configuration
Building upon the understanding of atomic number and electron arrangement, we now delve into the specifics of how Argon's 18 electrons are distributed around its nucleus. This distribution, known as the electron configuration, dictates Argon's chemical behavior and properties. Understanding this configuration is paramount to understanding Argon itself.
Deciphering the Notation: 1s² 2s² 2p⁶ 3s² 3p⁶
Argon's ground state electron configuration is represented as 1s² 2s² 2p⁶ 3s² 3p⁶. This notation, seemingly complex, is actually a concise map of electron locations. Each component provides crucial information about electron energy levels and spatial distribution.
Let's dissect this notation piece by piece. The numbers (1, 2, 3) represent the principal quantum number n, indicating the electron shell or energy level. Higher numbers signify shells further from the nucleus and thus higher energy. The letters (s, p) denote the subshells or atomic orbitals within each shell, each with a distinct shape and energy.
Finally, the superscripts (², ⁶) indicate the number of electrons occupying each subshell.
Electron Distribution in Detail
The configuration 1s² indicates that two electrons occupy the 1s subshell, which is the lowest energy subshell closest to the nucleus. This subshell can hold a maximum of two electrons.
The 2s² 2p⁶ segment signifies that the second shell (n=2) contains two electrons in the 2s subshell and six electrons in the 2p subshell. Recall that the p subshell can hold a maximum of six electrons.
Finally, 3s² 3p⁶ denotes that the third shell (n=3) similarly contains two electrons in the 3s subshell and six electrons in the 3p subshell.
This configuration showcases that the outermost shell of Argon is completely full.
The Significance of a Full Outermost Shell
Argon's electron configuration terminates with 3s² 3p⁶, indicating a completely filled outermost electron shell (the n=3 shell). This complete filling, also known as a closed shell, is the key to understanding Argon's inertness. This stable configuration minimizes Argon's tendency to participate in chemical reactions, setting it apart from elements with incomplete valence shells.
Shell-by-Shell Breakdown: A Detailed Look at Electron Distribution
Mapping the Electrons: Argon's Ground State Configuration Building upon the understanding of atomic number and electron arrangement, we now delve into the specifics of how Argon's 18 electrons are distributed around its nucleus. This distribution, known as the electron configuration, dictates Argon's chemical behavior and properties. Understanding...
Decoding the Electron Distribution
Argon's electron configuration, 1s² 2s² 2p⁶ 3s² 3p⁶, describes a meticulously organized arrangement. To truly grasp the stability of Argon, we need to dissect this configuration shell by shell, examining the occupancy and the capacity of each energy level. This detailed exploration reveals the underlying principles governing Argon's inertness.
The Innermost K Shell (n=1): A Complete 1s²
The innermost shell, denoted as the K shell or n=1, is the closest to the nucleus. This shell houses a single s orbital, capable of accommodating a maximum of two electrons.
Argon's K shell is completely filled with its two electrons occupying the 1s orbital (1s²). This complete filling contributes to the overall stability of the atom by effectively shielding the nucleus.
The L Shell (n=2): A Full Complement of 2s² 2p⁶
Moving outwards, the L shell (n=2) presents a more complex structure. This shell comprises one s orbital (2s) and three p orbitals (2p).
The 2s orbital can hold up to two electrons, while the three 2p orbitals can collectively accommodate six electrons.
Argon's L shell is fully occupied with two electrons in the 2s orbital and six electrons in the 2p orbitals (2s² 2p⁶), bringing the total electron count in the L shell to eight.
The M Shell (n=3): Achieving Octet Stability with 3s² 3p⁶
The M shell (n=3) is Argon's outermost, or valence, shell. This shell, similarly to the L shell, contains one s orbital (3s) and three p orbitals (3p).
Just as with the L shell, the 3s orbital can house two electrons and the three 3p orbitals can hold six electrons.
Argon's M shell accommodates two electrons in the 3s orbital and six electrons in the 3p orbitals (3s² 3p⁶). This configuration results in a total of eight electrons in the valence shell, adhering perfectly to the octet rule.
Maximum Electron Capacity and Argon's Compliance
Each electron shell possesses a specific maximum capacity for electrons, dictated by quantum mechanical principles. The first shell (K) can hold a maximum of 2 electrons.
The second shell (L) can hold a maximum of 8 electrons, and the third shell (M) can also hold a maximum of 8 electrons when it serves as the valence shell.
Argon's electron configuration precisely adheres to these limitations. Its K shell contains 2 electrons, its L shell contains 8 electrons, and its M shell, acting as the valence shell, also contains 8 electrons.
This optimized arrangement underscores Argon's inherent stability and its reluctance to participate in chemical reactions.
The Octet Rule and Noble Gas Stability: The Key to Argon's Inertness
Building upon the shell-by-shell breakdown of electron distribution, we now arrive at the crux of Argon's behavior: its profound inertness. This characteristic stems directly from its electron configuration and its adherence to the venerable octet rule, a fundamental principle governing chemical stability.
The Octet Rule: A Foundation of Chemical Stability
The octet rule, a cornerstone of chemical understanding, posits that atoms strive to achieve an electron configuration resembling that of the noble gases. These elements, residing in Group 18 of the periodic table, possess an inherent stability due to their full valence shells, typically containing eight electrons.
This "octet" of electrons in the outermost shell confers a state of minimal energy, making the atom exceptionally unreactive. Atoms readily gain, lose, or share electrons to attain this coveted configuration, driving the formation of chemical bonds.
Valence Electrons: Defining Reactivity
Valence electrons are the electrons residing in the outermost electron shell of an atom. These electrons are the key players in chemical bonding. They dictate how an atom interacts with other atoms.
For Argon, the story is simple. Its M shell (n=3) contains the 3s² 3p⁶ configuration, giving it a total of eight valence electrons. This complete octet is the reason for its stability. It's the foundation for Argon's aloofness in the chemical world.
Argon's Inertness: A Consequence of a Full Valence Shell
The presence of eight valence electrons in Argon's outermost shell fulfills the octet rule. This confers exceptional stability. The atom has no energetic driving force to gain, lose, or share electrons with other atoms.
This complete valence shell is the direct cause of Argon's inertness. It explains why Argon exists as a monatomic gas and doesn't readily form chemical bonds. It doesn't engage in chemical reactions under normal conditions.
This resistance to chemical interactions distinguishes it from most other elements. They are constantly seeking to achieve a more stable electron configuration through bonding. Argon, in contrast, is already content and self-sufficient. Its electron structure is perfect as it is.
Related Properties: Ionization Energy and Electronegativity
[The Octet Rule and Noble Gas Stability: The Key to Argon's Inertness Building upon the shell-by-shell breakdown of electron distribution, we now arrive at the crux of Argon's behavior: its profound inertness. This characteristic stems directly from its electron configuration and its adherence to the venerable octet rule, a fundamental principle governing the stability of atoms. However, to fully appreciate the implications of Argon's electron configuration, we must delve into two closely related properties: ionization energy and electronegativity.]
Ionization Energy: A Measure of Electron Binding
Ionization energy, a critical indicator of an atom's stability, quantifies the energy required to remove an electron from a gaseous atom.
Argon possesses a remarkably high ionization energy. This signifies that a substantial amount of energy is necessary to overcome the strong attraction between the nucleus and the outermost electrons.
But why is this the case?
The answer lies within its electron configuration.
Argon's filled valence shell (3s² 3p⁶) represents a particularly stable arrangement. Removing an electron disrupts this stability, requiring a significant energy input. This resistance to electron removal directly contributes to Argon's reluctance to participate in chemical reactions.
Electronegativity: The Quest for Electrons
Electronegativity, on the other hand, measures an atom's ability to attract electrons in a chemical bond. Unlike elements with a strong "hunger" for electrons, Argon exhibits exceptionally low electronegativity.
In essence, Argon has minimal desire to gain additional electrons.
This stems from its already complete valence shell. It has no "need" for extra electrons to achieve a stable configuration. Consequently, Argon demonstrates virtually no tendency to attract electrons from other atoms, reinforcing its inert nature.
The Electron Configuration Connection: Stability Confirmed
The high ionization energy and low electronegativity are not merely isolated properties; they are directly linked to Argon's stable electron configuration.
The filled valence shell, dictated by the 1s² 2s² 2p⁶ 3s² 3p⁶ arrangement, creates a scenario where removing or adding electrons is energetically unfavorable.
This energetic unfavorability, manifested as high ionization energy and low electronegativity, confirms and explains Argon's well-known chemical inertness. These properties underscore the profound influence of electron configuration on an element's fundamental behavior.
Why Electron Configuration Matters: Applications and Implications
Building upon the understanding of ionization energy and electronegativity, it's crucial to emphasize why elucidating Argon's electron configuration holds profound significance. This knowledge transcends mere academic curiosity; it is the cornerstone for comprehending the element's inherent properties and predictable behaviors.
A Foundation for Understanding Chemical Behavior
The electron configuration of Argon, particularly its filled outer shell, is not an isolated fact. It serves as a vital template for understanding its chemical properties. Argon's resistance to forming chemical bonds stems directly from its stable electron arrangement.
Its fully occupied 3s² 3p⁶ configuration negates the need to gain, lose, or share electrons, rendering it chemically inert under typical conditions. This inertness is why Argon finds applications in situations where an unreactive atmosphere is required.
Beyond Inertness: Applications of Noble Gases
Argon's electron configuration dictates its utility in various technological applications, illustrating the practical relevance of this seemingly theoretical knowledge.
Illuminating Applications: Argon in Lighting
One prominent example is in lighting. Argon is frequently used in incandescent light bulbs to prevent the filament from oxidizing at high temperatures. The inert atmosphere provided by Argon inhibits chemical reactions that would degrade the filament, thus extending the bulb's lifespan.
Preserving Materials: Argon in Welding
Furthermore, Argon is extensively employed as a shielding gas in welding. During welding processes, high temperatures can cause metals to react with oxygen and nitrogen in the air, leading to weakened welds and corrosion. Argon shields the welding area, preventing these undesirable reactions and ensuring the integrity of the weld.
The Broader Implications: Understanding Other Elements
Understanding Argon's electron configuration also provides a foundation for understanding the chemical bonding and behavior of other elements. By studying how Argon achieves stability, we gain insights into how other elements strive to attain similar stability through chemical reactions, electron sharing, or electron transfer.
The Quest for Stability: Chemical Bonding
Atoms combine to achieve stability. They attempt to arrange their electrons in a manner that resembles the stable electron configurations of noble gases. This pursuit of stability drives the formation of chemical bonds.
Elements like sodium and chlorine react to form sodium chloride (table salt), as it allows each element to attain the stable electron configuration of a noble gas. Sodium loses an electron to achieve the configuration of Neon, while chlorine gains an electron to achieve the configuration of Argon.
Predicting Reactivity: The Power of Electron Configuration
By knowing the electron configurations of elements, we can predict their potential reactivity and the types of chemical compounds they are likely to form. Elements with nearly full or nearly empty valence shells tend to be more reactive, as they have a stronger tendency to gain or lose electrons, respectively.
Therefore, the study of electron configuration is not merely an exercise in memorization; it is a key that unlocks a deeper understanding of chemical principles and the behavior of matter. It allows us to make predictions and design new materials with specific properties, solidifying its vital position in the scientific world.
Video: Argon Electron Configuration: Properties & Reactivity
Argon Electron Configuration: FAQs
Why is argon so unreactive?
Argon is highly unreactive due to its stable argon electron configuration. It possesses a full outermost electron shell (octet), meaning it doesn't readily gain, lose, or share electrons to form chemical bonds.
What is the electron configuration of argon?
The electron configuration of argon is 1s² 2s² 2p⁶ 3s² 3p⁶. This full outermost shell (3s² 3p⁶) gives argon its inert or noble gas properties. No need to form bonds to achieve stability.
Does argon form any compounds?
While generally inert, argon can form compounds under extreme conditions, such as with fluorine at very low temperatures. These compounds are rare and unstable because argon electron configuration strongly prefers remaining in the neutral, unbound, state.
How does argon's electron configuration relate to its uses?
Argon's unreactivity, dictated by its argon electron configuration, makes it useful in applications where an inert atmosphere is needed. Examples include welding, light bulbs, and protecting materials from oxidation.
So, there you have it! Argon, with its full outer shell and stable argon electron configuration, really is the cool cat of the noble gas family. It might not be setting the world on fire with reactivity, but its unique properties make it incredibly useful in a surprising number of applications. Who knew something so inert could be so important?